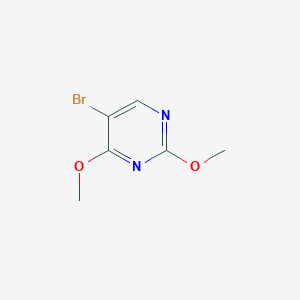
5-Bromo-2,4-dimethoxypyrimidine
Übersicht
Beschreibung
Synthesis Analysis
5-Bromo-2,4-dimethoxypyrimidine serves as a significant intermediate in the synthesis of various pyrimidine derivatives. The synthesis of pyrimidine compounds often involves multiple steps, including the bromination of precursor molecules. For example, the synthesis of 5-(4-bromophenyl)-4,6-dichloropyrimidine demonstrates a rapid synthetic method through three steps from commercially available precursors, achieving a total yield of 52.8% (Shan Hou et al., 2016).
Molecular Structure Analysis
The molecular structure of related pyrimidine derivatives has been extensively analyzed using techniques such as NMR spectroscopy, X-ray diffraction, and semiempirical MO calculations. For instance, the structure of a closely related compound, 5-Bromo-4,6-dimethoxy-4-(trichloromethyl)hexahydropyrimidin-2-one, was elucidated through these methods, providing insights into the diastereoisomerism and stability of the molecule (M. Martins et al., 1998).
Chemical Reactions and Properties
The chemical properties of this compound derivatives are characterized by their reactivity towards various chemical reactions, including condensation, bromination, and cross-coupling reactions. These reactions are pivotal for the synthesis of more complex molecules with potential pharmaceutical applications. For example, Suzuki cross-coupling reactions have been utilized to further diversify the structure of pyrimidine derivatives, demonstrating the versatility of these compounds as synthetic intermediates (Caifei Tang et al., 2014).
Wissenschaftliche Forschungsanwendungen
Anti-inflammatory Drug Development : Bromosubstituted 5-nitro-2,4(1H,3H)-pyrimidinediones, related to 5-Bromo-2,4-dimethoxypyrimidine, have shown potential for developing new anti-inflammatory drugs (Kinoshita et al., 1992).
DNA Synthesis Monitoring : Antibodies against bromodeoxyuridine (BrdU), a compound related to this compound, can recognize specific epitopes, aiding in accurate and sensitive DNA synthesis monitoring (Miller et al., 1986).
Inhibitors of Uridine Phosphorylase : Nucleoside analogues of uridine, including 5-bromo-, 5-iodo-, and 5-fluorouridines, have been synthesized and identified as competitive inhibitors of uridine phosphorylase in vitro (Abrams et al., 1981).
Chemical Synthesis : Palladium-catalyzed carbomethoxyvinylation and thienylation of 5-iodo(bromo)-2,4-dimethoxypyrimidine in water yield high yields of 5-(2-carbomethoxyvinyl)-2,4-dime (Bašnák et al., 1997).
Study of Cyclobutane Dimers : Photochemical studies of derivatives, including 5bromo2′-deoxyuridine, reveal new cyclobutane dimers with potential applications in nucleoside-mediated processes (Shetlar & Chung, 2012).
Antiviral Activity : 5-Substituted-2,4-diamino-6-[2-(phosphonomethoxy)ethoxy]pyrimidines, similar to this compound, show antiviral activity, particularly against HIV-1 (Hocková et al., 2003).
Pharmaceutical Synthesis : A novel route for synthesizing 4,5-difunctionalized uracils, applicable in pharmaceuticals like oxypurinol and emivirine, has been developed using a bromine/magnesium exchange reaction (Boudet & Knochel, 2006).
Cerebellar Neuroblast Studies : High or repeated doses of BrdU can impact cerebellar neuroblast proliferation and viability, highlighting the need for cautious interpretation of results in such studies (Martí-Clúa, 2021).
Nucleoside Synthesis : The Knoevenagel reaction has been used for the synthesis of various nucleoside analogues, demonstrating potential applications in nucleoside synthesis (Whale et al., 1992).
Safety and Hazards
5-Bromo-2,4-dimethoxypyrimidine is considered hazardous according to the 2012 OSHA Hazard Communication Standard (29 CFR 1910.1200) . It can cause skin irritation, serious eye irritation, and may cause respiratory irritation . It is recommended to wear protective gloves, protective clothing, eye protection, and face protection when handling this chemical .
Relevant Papers A paper titled “Synthesis, Molecular Docking, and ADME Prediction of Some Pyridine and Pyrimidine Derivatives as Anticolorectal Cancer Drugs” mentions that this compound is found to be most active against Thymidylate Synthase . The paper suggests that coupling these compounds with other known antineoplastic natural products could lead to more promising anticolorectal cancer compounds .
Wirkmechanismus
Target of Action
It is known to be used in palladium-catalyzed reactions , suggesting that it may interact with palladium complexes in biochemical systems.
Mode of Action
It is known to participate in palladium-catalyzed carbomethoxyvinylation and thienylation reactions . In these reactions, the compound likely interacts with its targets, leading to changes in their chemical structure.
Action Environment
Environmental factors can influence the action, efficacy, and stability of 5-Bromo-2,4-dimethoxypyrimidine. For instance, the compound’s reactivity in palladium-catalyzed reactions may be influenced by factors such as temperature, pH, and the presence of other chemicals .
Eigenschaften
IUPAC Name |
5-bromo-2,4-dimethoxypyrimidine | |
---|---|---|
Source | PubChem | |
URL | https://pubchem.ncbi.nlm.nih.gov | |
Description | Data deposited in or computed by PubChem | |
InChI |
InChI=1S/C6H7BrN2O2/c1-10-5-4(7)3-8-6(9-5)11-2/h3H,1-2H3 | |
Source | PubChem | |
URL | https://pubchem.ncbi.nlm.nih.gov | |
Description | Data deposited in or computed by PubChem | |
InChI Key |
QEZIMQMMEGPYTR-UHFFFAOYSA-N | |
Source | PubChem | |
URL | https://pubchem.ncbi.nlm.nih.gov | |
Description | Data deposited in or computed by PubChem | |
Canonical SMILES |
COC1=NC(=NC=C1Br)OC | |
Source | PubChem | |
URL | https://pubchem.ncbi.nlm.nih.gov | |
Description | Data deposited in or computed by PubChem | |
Molecular Formula |
C6H7BrN2O2 | |
Source | PubChem | |
URL | https://pubchem.ncbi.nlm.nih.gov | |
Description | Data deposited in or computed by PubChem | |
DSSTOX Substance ID |
DTXSID00292302 | |
Record name | 5-Bromo-2,4-dimethoxypyrimidine | |
Source | EPA DSSTox | |
URL | https://comptox.epa.gov/dashboard/DTXSID00292302 | |
Description | DSSTox provides a high quality public chemistry resource for supporting improved predictive toxicology. | |
Molecular Weight |
219.04 g/mol | |
Source | PubChem | |
URL | https://pubchem.ncbi.nlm.nih.gov | |
Description | Data deposited in or computed by PubChem | |
CAS RN |
56686-16-9 | |
Record name | 56686-16-9 | |
Source | DTP/NCI | |
URL | https://dtp.cancer.gov/dtpstandard/servlet/dwindex?searchtype=NSC&outputformat=html&searchlist=81442 | |
Description | The NCI Development Therapeutics Program (DTP) provides services and resources to the academic and private-sector research communities worldwide to facilitate the discovery and development of new cancer therapeutic agents. | |
Explanation | Unless otherwise indicated, all text within NCI products is free of copyright and may be reused without our permission. Credit the National Cancer Institute as the source. | |
Record name | 5-Bromo-2,4-dimethoxypyrimidine | |
Source | EPA DSSTox | |
URL | https://comptox.epa.gov/dashboard/DTXSID00292302 | |
Description | DSSTox provides a high quality public chemistry resource for supporting improved predictive toxicology. | |
Record name | 5-Bromo-2,4-dimethoxypyrimidine | |
Source | European Chemicals Agency (ECHA) | |
URL | https://echa.europa.eu/information-on-chemicals | |
Description | The European Chemicals Agency (ECHA) is an agency of the European Union which is the driving force among regulatory authorities in implementing the EU's groundbreaking chemicals legislation for the benefit of human health and the environment as well as for innovation and competitiveness. | |
Explanation | Use of the information, documents and data from the ECHA website is subject to the terms and conditions of this Legal Notice, and subject to other binding limitations provided for under applicable law, the information, documents and data made available on the ECHA website may be reproduced, distributed and/or used, totally or in part, for non-commercial purposes provided that ECHA is acknowledged as the source: "Source: European Chemicals Agency, http://echa.europa.eu/". Such acknowledgement must be included in each copy of the material. ECHA permits and encourages organisations and individuals to create links to the ECHA website under the following cumulative conditions: Links can only be made to webpages that provide a link to the Legal Notice page. | |
Retrosynthesis Analysis
AI-Powered Synthesis Planning: Our tool employs the Template_relevance Pistachio, Template_relevance Bkms_metabolic, Template_relevance Pistachio_ringbreaker, Template_relevance Reaxys, Template_relevance Reaxys_biocatalysis model, leveraging a vast database of chemical reactions to predict feasible synthetic routes.
One-Step Synthesis Focus: Specifically designed for one-step synthesis, it provides concise and direct routes for your target compounds, streamlining the synthesis process.
Accurate Predictions: Utilizing the extensive PISTACHIO, BKMS_METABOLIC, PISTACHIO_RINGBREAKER, REAXYS, REAXYS_BIOCATALYSIS database, our tool offers high-accuracy predictions, reflecting the latest in chemical research and data.
Strategy Settings
Precursor scoring | Relevance Heuristic |
---|---|
Min. plausibility | 0.01 |
Model | Template_relevance |
Template Set | Pistachio/Bkms_metabolic/Pistachio_ringbreaker/Reaxys/Reaxys_biocatalysis |
Top-N result to add to graph | 6 |
Feasible Synthetic Routes
Haftungsausschluss und Informationen zu In-Vitro-Forschungsprodukten
Bitte beachten Sie, dass alle Artikel und Produktinformationen, die auf BenchChem präsentiert werden, ausschließlich zu Informationszwecken bestimmt sind. Die auf BenchChem zum Kauf angebotenen Produkte sind speziell für In-vitro-Studien konzipiert, die außerhalb lebender Organismen durchgeführt werden. In-vitro-Studien, abgeleitet von dem lateinischen Begriff "in Glas", beinhalten Experimente, die in kontrollierten Laborumgebungen unter Verwendung von Zellen oder Geweben durchgeführt werden. Es ist wichtig zu beachten, dass diese Produkte nicht als Arzneimittel oder Medikamente eingestuft sind und keine Zulassung der FDA für die Vorbeugung, Behandlung oder Heilung von medizinischen Zuständen, Beschwerden oder Krankheiten erhalten haben. Wir müssen betonen, dass jede Form der körperlichen Einführung dieser Produkte in Menschen oder Tiere gesetzlich strikt untersagt ist. Es ist unerlässlich, sich an diese Richtlinien zu halten, um die Einhaltung rechtlicher und ethischer Standards in Forschung und Experiment zu gewährleisten.