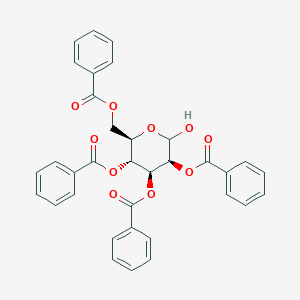
2,3,4,6-Tetra-O-benzoyl-D-mannopyranose
Übersicht
Beschreibung
2,3,4,6-Tetra-O-benzoyl-D-mannopyranose is a derivative of D-mannose, a naturally occurring sugar. This compound is characterized by the presence of four benzoyl groups attached to the hydroxyl groups at positions 2, 3, 4, and 6 of the mannopyranose ring. It is commonly used in organic synthesis and as a protective group in carbohydrate chemistry .
Vorbereitungsmethoden
Synthetic Routes and Reaction Conditions
2,3,4,6-Tetra-O-benzoyl-D-mannopyranose can be synthesized from D-mannose through a series of benzoylation reactions. The typical synthetic route involves the reaction of D-mannose with benzoyl chloride in the presence of a base such as pyridine. The reaction conditions usually include maintaining the reaction mixture at a low temperature to prevent decomposition and ensure high yield .
Industrial Production Methods
Industrial production of this compound follows similar synthetic routes but on a larger scale. The process involves the use of industrial-grade reagents and optimized reaction conditions to maximize yield and purity. The product is then purified through recrystallization or chromatography techniques .
Analyse Chemischer Reaktionen
Types of Reactions
2,3,4,6-Tetra-O-benzoyl-D-mannopyranose undergoes various chemical reactions, including:
Hydrolysis: The benzoyl groups can be hydrolyzed under acidic or basic conditions to yield D-mannose.
Oxidation: The compound can be oxidized to form corresponding carboxylic acids.
Substitution: The benzoyl groups can be substituted with other protective groups or functional groups under appropriate conditions.
Common Reagents and Conditions
Hydrolysis: Acidic or basic conditions, such as hydrochloric acid or sodium hydroxide.
Oxidation: Oxidizing agents like potassium permanganate or chromium trioxide.
Substitution: Reagents like sodium methoxide or other nucleophiles.
Major Products Formed
Hydrolysis: D-mannose
Oxidation: Carboxylic acids
Substitution: Various substituted mannopyranose derivatives.
Wissenschaftliche Forschungsanwendungen
Synthetic Organic Chemistry
Building Block for Carbohydrates
2,3,4,6-Tetra-O-benzoyl-D-mannopyranose serves as a versatile building block in the synthesis of complex carbohydrates and glycosides. This is crucial for developing new pharmaceuticals that require specific carbohydrate structures for biological activity.
- Case Study: In a study published by the Bulletin of the Chemical Society of Japan, researchers demonstrated the synthesis of various glycosides using this compound as a precursor, highlighting its utility in constructing complex carbohydrate frameworks .
Drug Development
Modifying Glycosylation Patterns
The compound is instrumental in modifying glycosylation patterns of drug candidates. This modification can enhance bioavailability and efficacy by targeting specific biological pathways.
- Example: Research has shown that altering glycosylation can significantly affect the pharmacokinetics and pharmacodynamics of therapeutic agents. For instance, studies involving antibody-drug conjugates (ADCs) have utilized this compound to improve targeting efficiency against cancer cells .
Biotechnology
Production of Glycoproteins
In biotechnology, this compound is used for preparing glycoproteins. These glycoproteins are essential for therapeutic proteins as they enhance stability and biological activity.
- Application Insight: The production of recombinant proteins often requires specific glycosylation patterns to ensure proper folding and function. This compound aids in achieving those patterns during the synthesis process .
Food Industry
Natural Sweeteners and Flavor Enhancers
The compound can be employed in the formulation of natural sweeteners and flavor enhancers. It provides an alternative to synthetic additives, catering to the growing demand for natural food products.
- Market Trend: The trend towards clean-label products has increased interest in using compounds like this compound as a natural sweetener in various food applications .
Research on Carbohydrate Chemistry
Studying Carbohydrate Interactions
This compound plays a critical role in research aimed at understanding carbohydrate interactions and functions within biological systems.
- Research Findings: Studies have utilized this compound to explore mechanisms of cellular recognition processes mediated by carbohydrates, which are vital for understanding disease mechanisms .
Wirkmechanismus
The mechanism of action of 2,3,4,6-Tetra-O-benzoyl-D-mannopyranose involves the protection of hydroxyl groups in D-mannose, preventing unwanted side reactions during chemical synthesis. The benzoyl groups can be selectively removed under specific conditions, allowing for the controlled modification of the mannopyranose ring. This selective protection and deprotection strategy is crucial in the synthesis of complex carbohydrates and glycoconjugates .
Vergleich Mit ähnlichen Verbindungen
Similar Compounds
- 2,3,4,6-Tetra-O-benzyl-D-mannopyranose
- 2,3,4,6-Tetra-O-acetyl-D-mannopyranose
- 2,3,4,6-Tetra-O-benzyl-D-glucopyranose
Uniqueness
2,3,4,6-Tetra-O-benzoyl-D-mannopyranose is unique due to its specific benzoyl protective groups, which offer distinct reactivity and stability compared to other protective groups like benzyl or acetyl. This uniqueness makes it particularly useful in specific synthetic applications where selective protection and deprotection are required .
Biologische Aktivität
2,3,4,6-Tetra-O-benzoyl-D-mannopyranose (TBM) is a derivative of D-mannose characterized by the presence of four benzoyl groups attached to the hydroxyl groups at positions 2, 3, 4, and 6 of the mannopyranose ring. This compound has garnered attention in various fields, particularly in organic synthesis and carbohydrate chemistry, due to its stability and reactivity. This article explores the biological activity of TBM, including its role in enzymatic reactions, potential therapeutic applications, and interactions with biological macromolecules.
TBM is synthesized through a series of acylation reactions that protect the hydroxyl groups of D-mannose. The presence of benzoyl groups enhances the compound's solubility in organic solvents and its stability during synthetic processes. Its molecular formula is with a molecular weight of approximately 596.59 g/mol .
Enzymatic Interactions
TBM serves as a substrate for various enzymes involved in carbohydrate metabolism. Notably, it participates in glycosylation reactions essential for synthesizing glycoproteins and glycolipids. Research indicates that TBM can influence biological pathways by modulating enzyme activity and facilitating carbohydrate-protein interactions.
Table 1: Enzymatic Reactions Involving TBM
Enzyme | Reaction Type | Product |
---|---|---|
Glycosyltransferases | Glycosylation | Glycoproteins |
Hydrolytic enzymes | Deacylation | D-mannopyranose |
Oxidative enzymes | Oxidation | Carboxylic acids |
Reductive enzymes | Reduction | D-mannopyranose |
Therapeutic Applications
The derivatives of TBM are being explored for their potential therapeutic applications. For instance, they have been studied for their roles in drug delivery systems and as precursors for synthesizing bioactive compounds. The ability to modulate immune responses and influence cell signaling pathways makes TBM a candidate for further research in pharmacology.
Case Studies
- Glycosylation Studies : A study demonstrated that TBM could be effectively used as a donor in glycosylation reactions to produce complex carbohydrate structures. The efficiency of TBM in these reactions highlights its utility in synthesizing biologically relevant glycosides .
- Immunomodulatory Effects : Research has indicated that compounds derived from TBM may exhibit immunomodulatory effects. These effects are crucial for developing new therapeutic agents targeting immune-related diseases .
The exact mechanism by which TBM exerts its biological effects is not fully understood; however, it is believed to involve the formation of oxonium intermediates during glycosylation reactions. This mechanism allows for the selective formation of glycosidic bonds between carbohydrates and proteins or other biomolecules .
Comparison with Similar Compounds
TBM can be compared with other benzoylated sugars, which share structural similarities but differ in their reactivity profiles:
Table 2: Comparison of Benzoylated Sugars
Compound Name | Structural Features | Unique Aspects |
---|---|---|
2,3,4-Tri-O-benzoyl-D-mannopyranose | Three benzoyl groups | Less sterically hindered than tetra-O-benzoyl form |
2-O-benzoyl-D-mannopyranose | One benzoyl group | More reactive due to fewer protections |
2,3-Di-O-benzoyl-D-mannopyranose | Two benzoyl groups | Intermediate reactivity |
2,3,4-Tri-O-acetyl-D-mannopyranose | Acetyl instead of benzoyl groups | Different reactivity profile |
The unique aspect of TBM lies in its stability and versatility due to the presence of four benzoyl groups, allowing selective reactions while maintaining structural integrity during synthetic processes.
Eigenschaften
IUPAC Name |
[(2R,3R,4S,5S)-3,4,5-tribenzoyloxy-6-hydroxyoxan-2-yl]methyl benzoate | |
---|---|---|
Source | PubChem | |
URL | https://pubchem.ncbi.nlm.nih.gov | |
Description | Data deposited in or computed by PubChem | |
InChI |
InChI=1S/C34H28O10/c35-30(22-13-5-1-6-14-22)40-21-26-27(42-31(36)23-15-7-2-8-16-23)28(43-32(37)24-17-9-3-10-18-24)29(34(39)41-26)44-33(38)25-19-11-4-12-20-25/h1-20,26-29,34,39H,21H2/t26-,27-,28+,29+,34?/m1/s1 | |
Source | PubChem | |
URL | https://pubchem.ncbi.nlm.nih.gov | |
Description | Data deposited in or computed by PubChem | |
InChI Key |
FCDYAJBVISGNLC-FUDYUEBSSA-N | |
Source | PubChem | |
URL | https://pubchem.ncbi.nlm.nih.gov | |
Description | Data deposited in or computed by PubChem | |
Canonical SMILES |
C1=CC=C(C=C1)C(=O)OCC2C(C(C(C(O2)O)OC(=O)C3=CC=CC=C3)OC(=O)C4=CC=CC=C4)OC(=O)C5=CC=CC=C5 | |
Source | PubChem | |
URL | https://pubchem.ncbi.nlm.nih.gov | |
Description | Data deposited in or computed by PubChem | |
Isomeric SMILES |
C1=CC=C(C=C1)C(=O)OC[C@@H]2[C@H]([C@@H]([C@@H](C(O2)O)OC(=O)C3=CC=CC=C3)OC(=O)C4=CC=CC=C4)OC(=O)C5=CC=CC=C5 | |
Source | PubChem | |
URL | https://pubchem.ncbi.nlm.nih.gov | |
Description | Data deposited in or computed by PubChem | |
Molecular Formula |
C34H28O10 | |
Source | PubChem | |
URL | https://pubchem.ncbi.nlm.nih.gov | |
Description | Data deposited in or computed by PubChem | |
DSSTOX Substance ID |
DTXSID80921103 | |
Record name | 2,3,4,6-Tetra-O-benzoylhexopyranose | |
Source | EPA DSSTox | |
URL | https://comptox.epa.gov/dashboard/DTXSID80921103 | |
Description | DSSTox provides a high quality public chemistry resource for supporting improved predictive toxicology. | |
Molecular Weight |
596.6 g/mol | |
Source | PubChem | |
URL | https://pubchem.ncbi.nlm.nih.gov | |
Description | Data deposited in or computed by PubChem | |
CAS No. |
113544-59-5 | |
Record name | 2,3,4,6-Tetra-O-benzoylhexopyranose | |
Source | EPA DSSTox | |
URL | https://comptox.epa.gov/dashboard/DTXSID80921103 | |
Description | DSSTox provides a high quality public chemistry resource for supporting improved predictive toxicology. | |
Retrosynthesis Analysis
AI-Powered Synthesis Planning: Our tool employs the Template_relevance Pistachio, Template_relevance Bkms_metabolic, Template_relevance Pistachio_ringbreaker, Template_relevance Reaxys, Template_relevance Reaxys_biocatalysis model, leveraging a vast database of chemical reactions to predict feasible synthetic routes.
One-Step Synthesis Focus: Specifically designed for one-step synthesis, it provides concise and direct routes for your target compounds, streamlining the synthesis process.
Accurate Predictions: Utilizing the extensive PISTACHIO, BKMS_METABOLIC, PISTACHIO_RINGBREAKER, REAXYS, REAXYS_BIOCATALYSIS database, our tool offers high-accuracy predictions, reflecting the latest in chemical research and data.
Strategy Settings
Precursor scoring | Relevance Heuristic |
---|---|
Min. plausibility | 0.01 |
Model | Template_relevance |
Template Set | Pistachio/Bkms_metabolic/Pistachio_ringbreaker/Reaxys/Reaxys_biocatalysis |
Top-N result to add to graph | 6 |
Feasible Synthetic Routes
Haftungsausschluss und Informationen zu In-Vitro-Forschungsprodukten
Bitte beachten Sie, dass alle Artikel und Produktinformationen, die auf BenchChem präsentiert werden, ausschließlich zu Informationszwecken bestimmt sind. Die auf BenchChem zum Kauf angebotenen Produkte sind speziell für In-vitro-Studien konzipiert, die außerhalb lebender Organismen durchgeführt werden. In-vitro-Studien, abgeleitet von dem lateinischen Begriff "in Glas", beinhalten Experimente, die in kontrollierten Laborumgebungen unter Verwendung von Zellen oder Geweben durchgeführt werden. Es ist wichtig zu beachten, dass diese Produkte nicht als Arzneimittel oder Medikamente eingestuft sind und keine Zulassung der FDA für die Vorbeugung, Behandlung oder Heilung von medizinischen Zuständen, Beschwerden oder Krankheiten erhalten haben. Wir müssen betonen, dass jede Form der körperlichen Einführung dieser Produkte in Menschen oder Tiere gesetzlich strikt untersagt ist. Es ist unerlässlich, sich an diese Richtlinien zu halten, um die Einhaltung rechtlicher und ethischer Standards in Forschung und Experiment zu gewährleisten.