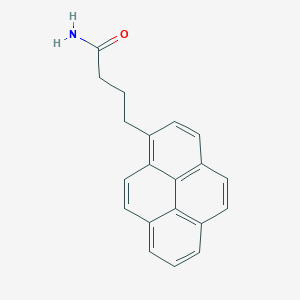
1-Pyrenbutanamid
Übersicht
Beschreibung
Synthesis Analysis
The synthesis of pyrene derivatives, including 1-Pyrenebutanamide, often involves complex reactions that aim to introduce specific functional groups to the pyrene core. For example, Sato et al. (2011) detailed the synthesis of unsymmetrically substituted pyrene derivatives through key precursors, highlighting the versatility in creating variously substituted pyrenes for different applications (Sato, Maeda, Mihara, & Iwasawa, 2011).
Molecular Structure Analysis
The molecular structure of pyrene derivatives plays a critical role in their chemical and physical properties. Niko et al. (2015) synthesized tetrasubstituted asymmetric pyrenes and examined their photophysical properties, demonstrating how structural modifications influence these properties (Niko, Sasaki, Narushima, Sharma, Vacha, & Konishi, 2015).
Chemical Reactions and Properties
Pyrenebutanamide and its derivatives undergo various chemical reactions, influencing their practical applications. Wrona-Piotrowicz et al. (2014) discussed the efficient synthesis of pyrene-1-carbothioamides and carboxamides, showcasing tunable solid-state fluorescence, which is pivotal in applications like fluorescent probes and organic electronics (Wrona-Piotrowicz, Zakrzewski, Métivier, Brosseau, Makal, & Woźniak, 2014).
Physical Properties Analysis
The physical properties of pyrene derivatives, such as solubility, melting point, and thermal stability, are significantly influenced by the nature of the substituent groups attached to the pyrene core. The synthesis and structural analysis of pyrene derivatives provide insights into how these properties can be tailored for specific needs.
Chemical Properties Analysis
The chemical properties of pyrene derivatives, including 1-Pyrenebutanamide, encompass a wide range of reactivities and interactions, depending on their functional groups. Studies like those by Davydov, Kumar, and Halpert (2002) on allosteric mechanisms in P450eryF probed with 1-pyrenebutanol, a related compound, highlight the intricate balance between structure and reactivity in biological systems (Davydov, Kumar, & Halpert, 2002).
Wissenschaftliche Forschungsanwendungen
Nanopartikel-Formulierung für bildgestützte Chirurgie
1-Pyrenbutanamid wird in der Formulierung von Nanopartikeln für die bildgestützte Chirurgie verwendet . Genauer gesagt, wird es bei der Herstellung von Aminopropyl-1-Pyrenbutanamid (PBA) verwendet, das an Hyaluronsäure (HA) konjugiert wird, um HA-PBA-Nanopartikel zu bilden . Diese Nanopartikel werden verwendet, um den intraoperativen Kontrast zu verbessern und so die Vollständigkeit der Tumorentfernung zu erhöhen .
Fluoreszierende Sonden zur Temperaturüberwachung
This compound wurde zur Herstellung von fluoreszierenden Sonden verwendet, die die Temperatur in organischen Lösungsmitteln präzise messen können . Dies ermöglicht nicht-invasive Messungen mit einer Genauigkeit von 1 °C .
Arzneimittel-Abgabesysteme
Die hydrophoben Wechselwirkungen von this compound ermöglichen die Einarbeitung von hydrophoben oder amphipathischen Molekülen . Diese Eigenschaft macht es nützlich für die Entwicklung von Arzneimittel-Abgabesystemen, insbesondere für die Abgabe von hydrophoben Arzneimitteln .
Tumorbilderung
Die durch this compound gebildeten Nanopartikel werden für die Bildgebung von soliden Tumoren verwendet . Sie haben gezeigt, dass sie den intraoperativen Kontrast verbessern und eine größere Anzahl kleiner, okkulter Läsionen als andere Methoden identifizieren .
Identifizierung der Brustwandinvasion
In einem Modell des späten Brustkrebsstadiums konnten durch this compound gebildete Nanopartikel die Brustwandinvasion identifizieren und das Wiederauftreten vorhersagen
Wirkmechanismus
Target of Action
1-Pyrenebutanamide, also known as PBA, is a water-soluble derivative of pyrene . It is primarily used in the formation of fluorescent probes for sensing applications . The primary target of PBA is the cucurbit10uril (Q10), a type of molecular container . PBA is encapsulated into Q10 in an aqueous solution to form a stable 1:1 host-guest inclusion complex .
Mode of Action
The mode of action of PBA involves the formation of a host-guest complex with Q10. This complex is formed through hydrophobic interactions . PBA interactions within the Q10 nanoparticle form hydrophobic domains , which allow for the incorporation of hydrophobic or amphiphilic molecules .
Pharmacokinetics
Its water-solubility suggests that it may have good bioavailability .
Result of Action
The primary result of PBA’s action is the formation of a fluorescent probe when complexed with Q10. This probe has been used for the selective recognition and determination of certain substances, such as dodine, among different pesticides . The addition of these substances to the PBA-Q10 complex results in a dramatic enhancement of fluorescence intensity at certain wavelengths .
Action Environment
The action of PBA is influenced by environmental factors. For instance, the formation of the PBA-Q10 complex and its fluorescence properties are dependent on the aqueous environment . Furthermore, the selectivity of the PBA-Q10
Eigenschaften
IUPAC Name |
4-pyren-1-ylbutanamide | |
---|---|---|
Source | PubChem | |
URL | https://pubchem.ncbi.nlm.nih.gov | |
Description | Data deposited in or computed by PubChem | |
InChI |
InChI=1S/C20H17NO/c21-18(22)6-2-3-13-7-8-16-10-9-14-4-1-5-15-11-12-17(13)20(16)19(14)15/h1,4-5,7-12H,2-3,6H2,(H2,21,22) | |
Source | PubChem | |
URL | https://pubchem.ncbi.nlm.nih.gov | |
Description | Data deposited in or computed by PubChem | |
InChI Key |
ADYCKJDIWFOVAR-UHFFFAOYSA-N | |
Source | PubChem | |
URL | https://pubchem.ncbi.nlm.nih.gov | |
Description | Data deposited in or computed by PubChem | |
Canonical SMILES |
C1=CC2=C3C(=C1)C=CC4=C(C=CC(=C43)C=C2)CCCC(=O)N | |
Source | PubChem | |
URL | https://pubchem.ncbi.nlm.nih.gov | |
Description | Data deposited in or computed by PubChem | |
Molecular Formula |
C20H17NO | |
Source | PubChem | |
URL | https://pubchem.ncbi.nlm.nih.gov | |
Description | Data deposited in or computed by PubChem | |
DSSTOX Substance ID |
DTXSID80390810 | |
Record name | 1-Pyrenebutanamide | |
Source | EPA DSSTox | |
URL | https://comptox.epa.gov/dashboard/DTXSID80390810 | |
Description | DSSTox provides a high quality public chemistry resource for supporting improved predictive toxicology. | |
Molecular Weight |
287.4 g/mol | |
Source | PubChem | |
URL | https://pubchem.ncbi.nlm.nih.gov | |
Description | Data deposited in or computed by PubChem | |
CAS RN |
71942-36-4 | |
Record name | 1-Pyrenebutanamide | |
Source | EPA DSSTox | |
URL | https://comptox.epa.gov/dashboard/DTXSID80390810 | |
Description | DSSTox provides a high quality public chemistry resource for supporting improved predictive toxicology. | |
Retrosynthesis Analysis
AI-Powered Synthesis Planning: Our tool employs the Template_relevance Pistachio, Template_relevance Bkms_metabolic, Template_relevance Pistachio_ringbreaker, Template_relevance Reaxys, Template_relevance Reaxys_biocatalysis model, leveraging a vast database of chemical reactions to predict feasible synthetic routes.
One-Step Synthesis Focus: Specifically designed for one-step synthesis, it provides concise and direct routes for your target compounds, streamlining the synthesis process.
Accurate Predictions: Utilizing the extensive PISTACHIO, BKMS_METABOLIC, PISTACHIO_RINGBREAKER, REAXYS, REAXYS_BIOCATALYSIS database, our tool offers high-accuracy predictions, reflecting the latest in chemical research and data.
Strategy Settings
Precursor scoring | Relevance Heuristic |
---|---|
Min. plausibility | 0.01 |
Model | Template_relevance |
Template Set | Pistachio/Bkms_metabolic/Pistachio_ringbreaker/Reaxys/Reaxys_biocatalysis |
Top-N result to add to graph | 6 |
Feasible Synthetic Routes
Q & A
Q1: How does 1-Pyrenebutanamide contribute to the development of tumor-targeted imaging agents?
A1: 1-Pyrenebutanamide can act as a hydrophobic building block in the self-assembly of nanoparticles designed for tumor imaging. [] In the highlighted study, 1-Pyrenebutanamide, conjugated to hyaluronic acid, facilitated the creation of nanoparticles that encapsulate Indocyanine green (ICG), a near-infrared fluorescent dye. These nanoparticles, termed NanoICG, demonstrated improved delivery of ICG to tumors in a mouse model. [] This suggests that incorporating 1-Pyrenebutanamide into the nanoparticle structure can enhance tumor targeting and imaging capabilities.
Q2: Are there any studies investigating the stability and behavior of 1-Pyrenebutanamide-based nanoparticles in biological environments?
A2: While the provided research [] doesn't delve into the detailed stability analysis of the 1-Pyrenebutanamide-based nanoparticles, it does indicate that these NanoICG particles exhibit quenched fluorescence that can be activated upon disassembly in a mixed solvent system. [] This suggests a degree of environmental responsiveness, which could be further investigated in the context of biological environments like blood serum or within tumor microenvironments. Future research could explore the stability, degradation, and potential release mechanisms of ICG from these nanoparticles in relevant biological settings to optimize their performance as tumor imaging agents.
Haftungsausschluss und Informationen zu In-Vitro-Forschungsprodukten
Bitte beachten Sie, dass alle Artikel und Produktinformationen, die auf BenchChem präsentiert werden, ausschließlich zu Informationszwecken bestimmt sind. Die auf BenchChem zum Kauf angebotenen Produkte sind speziell für In-vitro-Studien konzipiert, die außerhalb lebender Organismen durchgeführt werden. In-vitro-Studien, abgeleitet von dem lateinischen Begriff "in Glas", beinhalten Experimente, die in kontrollierten Laborumgebungen unter Verwendung von Zellen oder Geweben durchgeführt werden. Es ist wichtig zu beachten, dass diese Produkte nicht als Arzneimittel oder Medikamente eingestuft sind und keine Zulassung der FDA für die Vorbeugung, Behandlung oder Heilung von medizinischen Zuständen, Beschwerden oder Krankheiten erhalten haben. Wir müssen betonen, dass jede Form der körperlichen Einführung dieser Produkte in Menschen oder Tiere gesetzlich strikt untersagt ist. Es ist unerlässlich, sich an diese Richtlinien zu halten, um die Einhaltung rechtlicher und ethischer Standards in Forschung und Experiment zu gewährleisten.