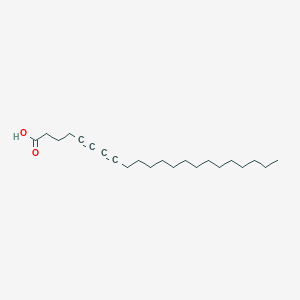
5,7-Docosadiynoic acid
Übersicht
Beschreibung
5,7-Docosadiynoic acid is a fluorescent molecule with a low molecular weight . This makes it ideal for use in a variety of strategies. This compound can be used as a fluorescent probe for the detection of membrane interactions and also has many potential applications in polymerized optical devices .
Synthesis Analysis
5,7-Docosadiynoic Acid is an amphiphilic molecule used in the synthesis of artificial cell membranes .
Molecular Structure Analysis
The molecular formula of 5,7-Docosadiynoic acid is C22H36O2 . The average mass is 332.520 Da and the monoisotopic mass is 332.271515 Da .
Physical And Chemical Properties Analysis
The physical and chemical properties of 5,7-Docosadiynoic acid are as follows :
Wissenschaftliche Forschungsanwendungen
Neuroscience Applications : DHA promotes neurogenesis both in vitro and in vivo, which could modulate hippocampal function regulated by neurogenesis (Kawakita, Hashimoto, & Shido, 2006). Additionally, DHA pre-administration has been found to beneficially affect the decline in learning ability in Alzheimer's disease model rats (Hashimoto et al., 2002).
Cardiovascular Health : DHA treatment inhibits intracellular Ca(2+) dynamics in vascular smooth muscle cells, contributing to its beneficial properties on cardiovascular disorders (Hirafuji et al., 2001).
Cancer Treatment and Prevention : DHA induces apoptosis in human MCF-7 breast cancer cells both in vitro and in vivo, which could aid in breast cancer treatment and prevention (Kang et al., 2010). It also enhances the anticancer effect of 5-fluorouracil (5-FU), suggesting a potential approach for cancer therapy (Zhuo et al., 2008).
Biochemical Synthesis : 5,7-Docosadiynoic acid is useful as an intermediate in the synthesis of arachidonic acid, poly-yn alcohols, and docosa-4,7,10,13,16,19-hexaynoic acid (Steen, Pabon, & Dorp, 2010).
Inflammatory and Immune Responses : DHA is a precursor to docosatrienes and 17S-series resolvins, which regulate inflammation and resolution events (Hong et al., 2003). It also attenuates immunoglobulin A nephropathy and IL-6 transcription in mice (Jia et al., 2004).
Eigenschaften
IUPAC Name |
docosa-5,7-diynoic acid | |
---|---|---|
Source | PubChem | |
URL | https://pubchem.ncbi.nlm.nih.gov | |
Description | Data deposited in or computed by PubChem | |
InChI |
InChI=1S/C22H36O2/c1-2-3-4-5-6-7-8-9-10-11-12-13-14-15-16-17-18-19-20-21-22(23)24/h2-14,19-21H2,1H3,(H,23,24) | |
Source | PubChem | |
URL | https://pubchem.ncbi.nlm.nih.gov | |
Description | Data deposited in or computed by PubChem | |
InChI Key |
KTCYROIQAAHHIE-UHFFFAOYSA-N | |
Source | PubChem | |
URL | https://pubchem.ncbi.nlm.nih.gov | |
Description | Data deposited in or computed by PubChem | |
Canonical SMILES |
CCCCCCCCCCCCCCC#CC#CCCCC(=O)O | |
Source | PubChem | |
URL | https://pubchem.ncbi.nlm.nih.gov | |
Description | Data deposited in or computed by PubChem | |
Molecular Formula |
C22H36O2 | |
Source | PubChem | |
URL | https://pubchem.ncbi.nlm.nih.gov | |
Description | Data deposited in or computed by PubChem | |
DSSTOX Substance ID |
DTXSID10407829 | |
Record name | 5,7-DOCOSADIYNOIC ACID | |
Source | EPA DSSTox | |
URL | https://comptox.epa.gov/dashboard/DTXSID10407829 | |
Description | DSSTox provides a high quality public chemistry resource for supporting improved predictive toxicology. | |
Molecular Weight |
332.5 g/mol | |
Source | PubChem | |
URL | https://pubchem.ncbi.nlm.nih.gov | |
Description | Data deposited in or computed by PubChem | |
Product Name |
5,7-Docosadiynoic acid | |
CAS RN |
178560-65-1 | |
Record name | 5,7-DOCOSADIYNOIC ACID | |
Source | EPA DSSTox | |
URL | https://comptox.epa.gov/dashboard/DTXSID10407829 | |
Description | DSSTox provides a high quality public chemistry resource for supporting improved predictive toxicology. | |
Synthesis routes and methods
Procedure details
Retrosynthesis Analysis
AI-Powered Synthesis Planning: Our tool employs the Template_relevance Pistachio, Template_relevance Bkms_metabolic, Template_relevance Pistachio_ringbreaker, Template_relevance Reaxys, Template_relevance Reaxys_biocatalysis model, leveraging a vast database of chemical reactions to predict feasible synthetic routes.
One-Step Synthesis Focus: Specifically designed for one-step synthesis, it provides concise and direct routes for your target compounds, streamlining the synthesis process.
Accurate Predictions: Utilizing the extensive PISTACHIO, BKMS_METABOLIC, PISTACHIO_RINGBREAKER, REAXYS, REAXYS_BIOCATALYSIS database, our tool offers high-accuracy predictions, reflecting the latest in chemical research and data.
Strategy Settings
Precursor scoring | Relevance Heuristic |
---|---|
Min. plausibility | 0.01 |
Model | Template_relevance |
Template Set | Pistachio/Bkms_metabolic/Pistachio_ringbreaker/Reaxys/Reaxys_biocatalysis |
Top-N result to add to graph | 6 |
Feasible Synthetic Routes
Haftungsausschluss und Informationen zu In-Vitro-Forschungsprodukten
Bitte beachten Sie, dass alle Artikel und Produktinformationen, die auf BenchChem präsentiert werden, ausschließlich zu Informationszwecken bestimmt sind. Die auf BenchChem zum Kauf angebotenen Produkte sind speziell für In-vitro-Studien konzipiert, die außerhalb lebender Organismen durchgeführt werden. In-vitro-Studien, abgeleitet von dem lateinischen Begriff "in Glas", beinhalten Experimente, die in kontrollierten Laborumgebungen unter Verwendung von Zellen oder Geweben durchgeführt werden. Es ist wichtig zu beachten, dass diese Produkte nicht als Arzneimittel oder Medikamente eingestuft sind und keine Zulassung der FDA für die Vorbeugung, Behandlung oder Heilung von medizinischen Zuständen, Beschwerden oder Krankheiten erhalten haben. Wir müssen betonen, dass jede Form der körperlichen Einführung dieser Produkte in Menschen oder Tiere gesetzlich strikt untersagt ist. Es ist unerlässlich, sich an diese Richtlinien zu halten, um die Einhaltung rechtlicher und ethischer Standards in Forschung und Experiment zu gewährleisten.