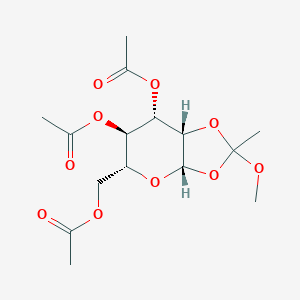
3,4,6-Tri-O-acetyl-beta-D-mannopyranose 1,2-(methyl orthoacetate)
- Klicken Sie auf QUICK INQUIRY, um ein Angebot von unserem Expertenteam zu erhalten.
- Mit qualitativ hochwertigen Produkten zu einem WETTBEWERBSFÄHIGEN Preis können Sie sich mehr auf Ihre Forschung konzentrieren.
Übersicht
Beschreibung
3,4,6-Tri-O-acetyl-beta-D-mannopyranose 1,2-(methyl orthoacetate), also known as 3,4,6-Tri-O-acetyl-beta-D-mannopyranose 1,2-(methyl orthoacetate), is a useful research compound. Its molecular formula is C15H22O10 and its molecular weight is 362.33 g/mol. The purity is usually 95%.
BenchChem offers high-quality 3,4,6-Tri-O-acetyl-beta-D-mannopyranose 1,2-(methyl orthoacetate) suitable for many research applications. Different packaging options are available to accommodate customers' requirements. Please inquire for more information about 3,4,6-Tri-O-acetyl-beta-D-mannopyranose 1,2-(methyl orthoacetate) including the price, delivery time, and more detailed information at info@benchchem.com.
Wirkmechanismus
Target of Action
It is known to be used in the preparation of acetobromomannose , which suggests that it may interact with enzymes or receptors that recognize or process mannose derivatives.
Biochemical Pathways
Given its role as a precursor in the synthesis of acetobromomannose , it may be involved in pathways related to carbohydrate metabolism or cell surface glycosylation.
Result of Action
As a precursor to acetobromomannose , its effects would likely be related to the functions of this compound, which could include roles in cell-cell interaction, immune response, or pathogen recognition.
Action Environment
The action, efficacy, and stability of 3,4,6-Tri-O-acetyl-beta-D-mannopyranose 1,2-(methyl orthoacetate) would be influenced by various environmental factors . These could include pH, temperature, and the presence of other molecules that can interact with mannose derivatives.
Biochemische Analyse
Biochemical Properties
The biochemical properties of 3,4,6-Tri-O-acetyl-beta-D-mannopyranose 1,2-(methyl orthoacetate) are not fully understood. It is known to interact with various enzymes, proteins, and other biomolecules. For instance, it has been used in the preparation of acetobromomannose , suggesting that it may interact with enzymes involved in carbohydrate metabolism.
Molecular Mechanism
It is known to be a precursor in the preparation of acetobromomannose , suggesting that it may undergo enzymatic reactions to form this compound
Metabolic Pathways
It is known to be a precursor in the preparation of acetobromomannose , suggesting that it may be involved in carbohydrate metabolism
Biologische Aktivität
3,4,6-Tri-O-acetyl-beta-D-mannopyranose 1,2-(methyl orthoacetate) is a derivative of mannose, a sugar that plays a significant role in various biological processes. This compound is notable for its potential applications in glycosylation reactions and as a precursor in the synthesis of more complex carbohydrates. Understanding its biological activity is crucial for its application in medicinal chemistry and biochemistry.
- Chemical Formula : C₁₃H₁₈O₇
- CAS Number : 4435-05-6
- Molecular Weight : 286.28 g/mol
Antimicrobial Properties
Research indicates that derivatives of mannose, including 3,4,6-Tri-O-acetyl-beta-D-mannopyranose, exhibit antimicrobial activity. For example, studies have shown that mannose derivatives can inhibit the growth of various bacteria and fungi. This activity is often attributed to their ability to interfere with microbial adhesion to host cells.
Glycosylation Reactions
3,4,6-Tri-O-acetyl-beta-D-mannopyranose is frequently used as a glycosyl donor in synthetic organic chemistry. Its acetyl groups serve as protecting groups that can be selectively removed during the synthesis of glycosides. This property enhances its utility in the preparation of complex carbohydrates and glycoconjugates.
Case Studies
-
Synthesis of Glycoconjugates :
A study demonstrated the successful use of 3,4,6-Tri-O-acetyl-beta-D-mannopyranose in synthesizing glycoproteins through glycosylation reactions. The compound was employed to attach mannose residues to peptide backbones, enhancing the biological activity of the resulting conjugates. -
Antiviral Activity :
In vitro studies have shown that certain mannose derivatives can inhibit viral replication. For instance, derivatives similar to 3,4,6-Tri-O-acetyl-beta-D-mannopyranose have been tested against HIV and demonstrated potential as antiviral agents by blocking viral entry into host cells.
The biological activity of 3,4,6-Tri-O-acetyl-beta-D-mannopyranose can be attributed to its structural characteristics:
- Molecular Interactions : The acetyl groups enhance solubility and facilitate interactions with biological macromolecules.
- Inhibition of Adhesion : By mimicking host cell sugars, mannose derivatives can prevent pathogens from adhering to host tissues.
Eigenschaften
CAS-Nummer |
4435-05-6 |
---|---|
Molekularformel |
C15H22O10 |
Molekulargewicht |
362.33 g/mol |
IUPAC-Name |
[(3aS,5R,6R,7S,7aS)-6,7-diacetyloxy-2-methoxy-2-methyl-5,6,7,7a-tetrahydro-3aH-[1,3]dioxolo[4,5-b]pyran-5-yl]methyl acetate |
InChI |
InChI=1S/C15H22O10/c1-7(16)20-6-10-11(21-8(2)17)12(22-9(3)18)13-14(23-10)25-15(4,19-5)24-13/h10-14H,6H2,1-5H3/t10-,11-,12+,13+,14+,15?/m1/s1 |
InChI-Schlüssel |
AUVGRVGPAIFPSA-PEONVUOVSA-N |
SMILES |
CC(=O)OCC1C(C(C2C(O1)OC(O2)(C)O)OC(=O)C)OC(=O)C |
Isomerische SMILES |
CC(=O)OC[C@@H]1[C@H]([C@@H]([C@H]2[C@@H](O1)OC(O2)(C)OC)OC(=O)C)OC(=O)C |
Kanonische SMILES |
CC(=O)OCC1C(C(C2C(O1)OC(O2)(C)OC)OC(=O)C)OC(=O)C |
Synonyme |
1,2-O-(1-Methoxyethylidene)-β-D-mannopyranose Triacetate; |
Herkunft des Produkts |
United States |
Haftungsausschluss und Informationen zu In-Vitro-Forschungsprodukten
Bitte beachten Sie, dass alle Artikel und Produktinformationen, die auf BenchChem präsentiert werden, ausschließlich zu Informationszwecken bestimmt sind. Die auf BenchChem zum Kauf angebotenen Produkte sind speziell für In-vitro-Studien konzipiert, die außerhalb lebender Organismen durchgeführt werden. In-vitro-Studien, abgeleitet von dem lateinischen Begriff "in Glas", beinhalten Experimente, die in kontrollierten Laborumgebungen unter Verwendung von Zellen oder Geweben durchgeführt werden. Es ist wichtig zu beachten, dass diese Produkte nicht als Arzneimittel oder Medikamente eingestuft sind und keine Zulassung der FDA für die Vorbeugung, Behandlung oder Heilung von medizinischen Zuständen, Beschwerden oder Krankheiten erhalten haben. Wir müssen betonen, dass jede Form der körperlichen Einführung dieser Produkte in Menschen oder Tiere gesetzlich strikt untersagt ist. Es ist unerlässlich, sich an diese Richtlinien zu halten, um die Einhaltung rechtlicher und ethischer Standards in Forschung und Experiment zu gewährleisten.