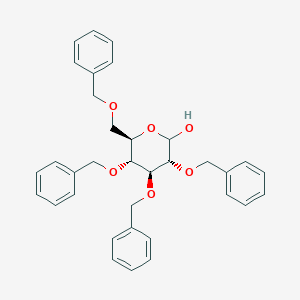
2,3,4,6-Tetra-O-benzyl-D-glucopyranose
Übersicht
Beschreibung
2,3,4,6-Tetra-O-benzyl-D-glucopyranose is a derivative of D-glucopyranose, which is a form of glucose. This compound is characterized by the presence of four benzyl groups attached to the hydroxyl groups at positions 2, 3, 4, and 6 of the glucopyranose ring. It is often used as an intermediate in the synthesis of various pharmaceutical compounds, including those for the treatment of Alzheimer’s disease, diabetes, and cancer .
Wissenschaftliche Forschungsanwendungen
2,3,4,6-Tetra-O-benzyl-D-glucopyranose is widely used in scientific research due to its versatility as an intermediate. Some of its applications include:
Chemistry: Used in the synthesis of complex carbohydrates and glycosylation reactions.
Biology: Serves as a building block for the synthesis of biologically active molecules.
Medicine: Intermediate in the synthesis of drugs for treating Alzheimer’s disease, diabetes, and cancer.
Industry: Used in the production of various pharmaceutical intermediates and fine chemicals
Wirkmechanismus
Target of Action
2,3,4,6-Tetra-O-benzyl-D-glucopyranose is primarily used as a reagent in organic synthesis . It is utilized for the preparation of α-glucopyranosyl chloride and 1-C-α-D-glucopyranosyl derivatives . These derivatives can be further used in the synthesis of important D-glucopyranosyl derivatives for glucosylation and other reactions .
Mode of Action
The compound acts as an intermediate for glucosylation couplings . It can be converted into this compound trichloroacetimidate using trichloroacetonitrile in the presence of a base such as potassium carbonate and DBU .
Biochemical Pathways
It is known that the compound plays a crucial role in the synthesis of glucosylated derivatives .
Result of Action
The primary result of the action of this compound is the production of α-glucopyranosyl chloride and 1-C-α-D-glucopyranosyl derivatives . These derivatives can be further used in the synthesis of important D-glucopyranosyl derivatives for glucosylation and other reactions .
Action Environment
The action of this compound is influenced by the conditions of the reaction in which it is used. For instance, the conversion of the compound into this compound trichloroacetimidate requires the presence of trichloroacetonitrile and a base . The stability and efficacy of the compound can be affected by factors such as temperature, pH, and the presence of other reactants.
Vorbereitungsmethoden
Synthetic Routes and Reaction Conditions
The synthesis of 2,3,4,6-Tetra-O-benzyl-D-glucopyranose typically involves the protection of the hydroxyl groups of D-glucopyranose with benzyl groups. One common method involves dissolving 2,3,4,6-tetra-O-benzyl-β-D-glucopyranose in acetone and adding N-bromosuccinimide (NBS) at 25°C. The reaction mixture is then subjected to reduced pressure to evaporate most of the acetone, followed by the addition of hydrochloric acid and cooling to precipitate the product .
Industrial Production Methods
In an industrial setting, the production of this compound may involve similar synthetic routes but on a larger scale. The use of automated reactors and precise control of reaction conditions ensures high yield and purity of the product. The compound is typically stored at low temperatures to maintain its stability .
Analyse Chemischer Reaktionen
Types of Reactions
2,3,4,6-Tetra-O-benzyl-D-glucopyranose undergoes various chemical reactions, including:
Oxidation: The compound can be oxidized to form glucosyl derivatives.
Reduction: Reduction reactions can be used to remove the benzyl protecting groups.
Substitution: The benzyl groups can be substituted with other functional groups to form different derivatives.
Common Reagents and Conditions
Oxidation: Common oxidizing agents include N-bromosuccinimide (NBS) and other halogen-based reagents.
Reduction: Catalytic hydrogenation is often used to remove benzyl groups.
Substitution: Various nucleophiles can be used to substitute the benzyl groups under appropriate conditions.
Major Products Formed
The major products formed from these reactions include α-glucopyranosyl chloride and 1-C-α-D-glucopyranosyl derivatives, which are important intermediates in the synthesis of other pharmaceutical compounds .
Vergleich Mit ähnlichen Verbindungen
Similar Compounds
2,3,4,6-Tetra-O-benzyl-D-galactopyranose: Similar structure but derived from galactose.
1,2,3,4-Tetra-O-acetyl-β-D-glucopyranose: Acetyl groups instead of benzyl groups.
2,3,4,6-Tetra-O-acetyl-D-galactopyranose: Acetyl groups and derived from galactose.
Uniqueness
2,3,4,6-Tetra-O-benzyl-D-glucopyranose is unique due to its specific benzyl protection, which provides stability and selectivity in chemical reactions. This makes it particularly valuable in the synthesis of complex molecules where precise control over reaction conditions is required .
Eigenschaften
IUPAC Name |
(3R,4S,5R,6R)-3,4,5-tris(phenylmethoxy)-6-(phenylmethoxymethyl)oxan-2-ol | |
---|---|---|
Source | PubChem | |
URL | https://pubchem.ncbi.nlm.nih.gov | |
Description | Data deposited in or computed by PubChem | |
InChI |
InChI=1S/C34H36O6/c35-34-33(39-24-29-19-11-4-12-20-29)32(38-23-28-17-9-3-10-18-28)31(37-22-27-15-7-2-8-16-27)30(40-34)25-36-21-26-13-5-1-6-14-26/h1-20,30-35H,21-25H2/t30-,31-,32+,33-,34?/m1/s1 | |
Source | PubChem | |
URL | https://pubchem.ncbi.nlm.nih.gov | |
Description | Data deposited in or computed by PubChem | |
InChI Key |
OGOMAWHSXRDAKZ-BKJHVTENSA-N | |
Source | PubChem | |
URL | https://pubchem.ncbi.nlm.nih.gov | |
Description | Data deposited in or computed by PubChem | |
Canonical SMILES |
C1=CC=C(C=C1)COCC2C(C(C(C(O2)O)OCC3=CC=CC=C3)OCC4=CC=CC=C4)OCC5=CC=CC=C5 | |
Source | PubChem | |
URL | https://pubchem.ncbi.nlm.nih.gov | |
Description | Data deposited in or computed by PubChem | |
Isomeric SMILES |
C1=CC=C(C=C1)COC[C@@H]2[C@H]([C@@H]([C@H](C(O2)O)OCC3=CC=CC=C3)OCC4=CC=CC=C4)OCC5=CC=CC=C5 | |
Source | PubChem | |
URL | https://pubchem.ncbi.nlm.nih.gov | |
Description | Data deposited in or computed by PubChem | |
Molecular Formula |
C34H36O6 | |
Source | PubChem | |
URL | https://pubchem.ncbi.nlm.nih.gov | |
Description | Data deposited in or computed by PubChem | |
DSSTOX Substance ID |
DTXSID001232816 | |
Record name | 2,3,4,6-Tetrakis-O-(phenylmethyl)-D-glucopyranose | |
Source | EPA DSSTox | |
URL | https://comptox.epa.gov/dashboard/DTXSID001232816 | |
Description | DSSTox provides a high quality public chemistry resource for supporting improved predictive toxicology. | |
Molecular Weight |
540.6 g/mol | |
Source | PubChem | |
URL | https://pubchem.ncbi.nlm.nih.gov | |
Description | Data deposited in or computed by PubChem | |
CAS No. |
4132-28-9 | |
Record name | 2,3,4,6-Tetrakis-O-(phenylmethyl)-D-glucopyranose | |
Source | CAS Common Chemistry | |
URL | https://commonchemistry.cas.org/detail?cas_rn=4132-28-9 | |
Description | CAS Common Chemistry is an open community resource for accessing chemical information. Nearly 500,000 chemical substances from CAS REGISTRY cover areas of community interest, including common and frequently regulated chemicals, and those relevant to high school and undergraduate chemistry classes. This chemical information, curated by our expert scientists, is provided in alignment with our mission as a division of the American Chemical Society. | |
Explanation | The data from CAS Common Chemistry is provided under a CC-BY-NC 4.0 license, unless otherwise stated. | |
Record name | 2,3,4,6-Tetrakis-O-(phenylmethyl)-D-glucopyranose | |
Source | EPA DSSTox | |
URL | https://comptox.epa.gov/dashboard/DTXSID001232816 | |
Description | DSSTox provides a high quality public chemistry resource for supporting improved predictive toxicology. | |
Record name | D-Glucopyranose, 2,3,4,6-tetrakis-O-(phenylmethyl) | |
Source | European Chemicals Agency (ECHA) | |
URL | https://echa.europa.eu/information-on-chemicals | |
Description | The European Chemicals Agency (ECHA) is an agency of the European Union which is the driving force among regulatory authorities in implementing the EU's groundbreaking chemicals legislation for the benefit of human health and the environment as well as for innovation and competitiveness. | |
Explanation | Use of the information, documents and data from the ECHA website is subject to the terms and conditions of this Legal Notice, and subject to other binding limitations provided for under applicable law, the information, documents and data made available on the ECHA website may be reproduced, distributed and/or used, totally or in part, for non-commercial purposes provided that ECHA is acknowledged as the source: "Source: European Chemicals Agency, http://echa.europa.eu/". Such acknowledgement must be included in each copy of the material. ECHA permits and encourages organisations and individuals to create links to the ECHA website under the following cumulative conditions: Links can only be made to webpages that provide a link to the Legal Notice page. | |
Synthesis routes and methods
Procedure details
Retrosynthesis Analysis
AI-Powered Synthesis Planning: Our tool employs the Template_relevance Pistachio, Template_relevance Bkms_metabolic, Template_relevance Pistachio_ringbreaker, Template_relevance Reaxys, Template_relevance Reaxys_biocatalysis model, leveraging a vast database of chemical reactions to predict feasible synthetic routes.
One-Step Synthesis Focus: Specifically designed for one-step synthesis, it provides concise and direct routes for your target compounds, streamlining the synthesis process.
Accurate Predictions: Utilizing the extensive PISTACHIO, BKMS_METABOLIC, PISTACHIO_RINGBREAKER, REAXYS, REAXYS_BIOCATALYSIS database, our tool offers high-accuracy predictions, reflecting the latest in chemical research and data.
Strategy Settings
Precursor scoring | Relevance Heuristic |
---|---|
Min. plausibility | 0.01 |
Model | Template_relevance |
Template Set | Pistachio/Bkms_metabolic/Pistachio_ringbreaker/Reaxys/Reaxys_biocatalysis |
Top-N result to add to graph | 6 |
Feasible Synthetic Routes
Q1: What is the molecular formula and weight of 2,3,4,6-Tetra-O-benzyl-D-glucopyranose?
A1: The molecular formula is C34H38O6, and its molecular weight is 542.66 g/mol.
Q2: Is there any spectroscopic data available for this compound?
A2: Yes, multiple studies utilize 1H NMR spectroscopy and mass spectrometry to characterize the compound and its derivatives. [, , , , , ]
Q3: What makes this compound a valuable starting material in organic synthesis?
A3: Its readily available benzyl protecting groups allow for selective deprotection and manipulation of the hydroxyl groups, enabling the synthesis of various carbohydrate derivatives. [, , , , , , , , , , , ]
Q4: Can you provide examples of compounds synthesized using this compound as a starting material?
A4: Researchers have successfully synthesized various compounds, including:
- (+)-Nojirimycin: A potent glucosidase inhibitor synthesized from this compound through a multi-step process involving oxidation, reduction, and deprotection steps. []
- Ferrocenyl carbohydrate conjugates: These conjugates, synthesized by coupling reactions with ferrocene carbonyl chloride, show potential as antimalarial agents. []
- C-D-glucopyranosyl derivatives: Synthesized by reacting the compound, activated by trifluoroacetic anhydride, with silyl enol ethers, allylsilane, or activated aromatic nucleophiles. []
- C-glucopyranosylproline hybrids: These hybrids, incorporated into peptides, allow for controlled variation of prolyl trans/cis-amide rotamer populations. []
- l-ido-1-deoxynojirimycin derivatives: These were synthesized via a three-step process involving reductive amination, "borrowing hydrogen" under neat conditions, and debenzylation. []
Q5: What are some of the key reactions this compound can undergo?
A5: this compound can undergo various reactions, including:
- Glycosylation reactions: It acts as a glycosyl donor in the synthesis of both α- and β-glycosides under specific catalytic conditions. [, , , , ]
- Wittig-Horner reactions: This reaction allows for the synthesis of both α- and β-glycosyl acetates. [, ]
- Reductive amination: This reaction pathway facilitates the introduction of amine functionalities, essential for synthesizing iminosugars like l-ido-1-deoxynojirimycin derivatives. []
- Conversion to L-sorbopyranose: This transformation can be achieved using bromomagnesium salts in dichloromethane. [, ]
Q6: What role does stereoselectivity play in reactions involving this compound?
A6: Stereoselectivity is crucial in utilizing this compound. For instance:
- C-glucopyranosyl derivatization: Reactions with silyl enol ethers or allylsilane yield α-anomers, while activated aromatic nucleophiles result in β-anomers. []
- C-allylation: Reactions with allyltrimethylsilane in the presence of trimethylsilyl trifluoromethanesulfonate predominantly yield α-C-allylated C-alkyl glucopyranosides. [, ]
- Mercuricyclisation: This reaction offers a stereospecific route to 1,5-trans (α-D) C-glucopyranosyl derivatives. []
Q7: How does the choice of catalyst influence the outcome of reactions with this compound?
A7: Different catalysts can significantly impact the stereochemical outcome:
- Lewis acids: The choice of Lewis acid catalyst influences the stereoselectivity in C-glycosylation reactions, leading to either α- or β-anomers. [, ]
- Tin-mediated alkylation: This approach allows for the introduction of specific protecting groups, facilitating the synthesis of desired carbohydrate derivatives. []
- Combination catalysts: Using a combination of SnCl4 and AgClO4 leads to α-glucoside formation, while SiCl4 and AgClO4 favor β-glucoside formation. []
Q8: Is there information available about the stability of this compound under different conditions?
A8: While specific stability data is limited in the provided research, the benzyl protecting groups offer stability during various synthetic transformations. Deprotection typically requires specific conditions, suggesting relative stability under standard storage conditions. [, , ]
Haftungsausschluss und Informationen zu In-Vitro-Forschungsprodukten
Bitte beachten Sie, dass alle Artikel und Produktinformationen, die auf BenchChem präsentiert werden, ausschließlich zu Informationszwecken bestimmt sind. Die auf BenchChem zum Kauf angebotenen Produkte sind speziell für In-vitro-Studien konzipiert, die außerhalb lebender Organismen durchgeführt werden. In-vitro-Studien, abgeleitet von dem lateinischen Begriff "in Glas", beinhalten Experimente, die in kontrollierten Laborumgebungen unter Verwendung von Zellen oder Geweben durchgeführt werden. Es ist wichtig zu beachten, dass diese Produkte nicht als Arzneimittel oder Medikamente eingestuft sind und keine Zulassung der FDA für die Vorbeugung, Behandlung oder Heilung von medizinischen Zuständen, Beschwerden oder Krankheiten erhalten haben. Wir müssen betonen, dass jede Form der körperlichen Einführung dieser Produkte in Menschen oder Tiere gesetzlich strikt untersagt ist. Es ist unerlässlich, sich an diese Richtlinien zu halten, um die Einhaltung rechtlicher und ethischer Standards in Forschung und Experiment zu gewährleisten.