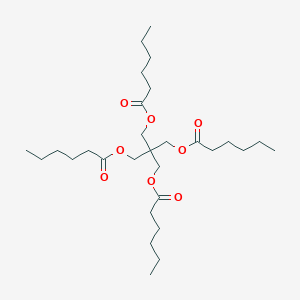
Pentaerythritoltetrahexanoat
Übersicht
Beschreibung
Pentaerythritol tetrahexanoate (PEH) is a synthetic ester of pentaerythritol, a polyol compound. It is a white, waxy solid that is insoluble in water, with a melting point of about 90 °C. PEH is used in a variety of applications, including as a plasticizer, lubricant, and surfactant. It is also used as an intermediate in the synthesis of other compounds.
Wissenschaftliche Forschungsanwendungen
Medizinische Therapien
Pentaerythritoltetrahexanoat wird in medizinischen Therapien eingesetzt, um die Symptombelastung bei chronisch stabiler Angina pectoris zu verringern . Dies ist besonders relevant für diejenigen, die keine Revaskularisationsmöglichkeiten mehr haben . Es scheint nicht die gleichen Toleranz-induzierenden Eigenschaften wie andere Nitrate aufzuweisen .
Gewebetechnik
This compound wird im Bereich der Gewebetechnik eingesetzt . Synthetische Biomaterialien, die zu porösen Gerüsten strukturiert werden können, um das Zellwachstum zu unterstützen, haben eine Rolle bei der Entwicklung dieses Bereichs gespielt . Die Morphologie zeigte ein vielversprechendes Ergebnis von Poly (Pentaerythritoltetrakis (3-Mercaptopropionat)/Dipentaerythritolpenta-/hexa-acrylat)HIPEs mit variierender Hypermer B246-Tensidkonzentration, die zu einer Vergrösserung der Porengrösse führte .
Photolumineszenz
This compound wurde auf seine Photolumineszenzeigenschaften untersucht . Photolumineszenz ist die Emission von Licht von jeder Form von Materie nach der Absorption von Photonen .
Klebstoffanwendungen
This compound wurde in Klebstoffanwendungen eingesetzt . Klebstoffe sind Stoffe, die auf eine oder beide Oberflächen von zwei getrennten Gegenständen aufgetragen werden, die diese miteinander verbinden und ihre Trennung verhindern
Wirkmechanismus
Target of Action
Pentaerythritol tetrahexanoate (PEH) is a synthetic ester derived from pentaerythritol, a polyol compound
Pharmacokinetics
They are typically metabolized in the liver by esterases, enzymes that catalyze the hydrolysis of esters .
Action Environment
PEH is a versatile compound with a balance between hydrophobic and hydrophilic properties, influencing its solubility and compatibility with various polymers and resins . This makes it valuable in various applications, serving as a plasticizer, lubricant, and surfactant . Its action, efficacy, and stability can be influenced by environmental factors such as temperature, pH, and the presence of other chemicals .
Eigenschaften
IUPAC Name |
[3-hexanoyloxy-2,2-bis(hexanoyloxymethyl)propyl] hexanoate | |
---|---|---|
Source | PubChem | |
URL | https://pubchem.ncbi.nlm.nih.gov | |
Description | Data deposited in or computed by PubChem | |
InChI |
InChI=1S/C29H52O8/c1-5-9-13-17-25(30)34-21-29(22-35-26(31)18-14-10-6-2,23-36-27(32)19-15-11-7-3)24-37-28(33)20-16-12-8-4/h5-24H2,1-4H3 | |
Source | PubChem | |
URL | https://pubchem.ncbi.nlm.nih.gov | |
Description | Data deposited in or computed by PubChem | |
InChI Key |
AOZDHFFNBZAHJF-UHFFFAOYSA-N | |
Source | PubChem | |
URL | https://pubchem.ncbi.nlm.nih.gov | |
Description | Data deposited in or computed by PubChem | |
Canonical SMILES |
CCCCCC(=O)OCC(COC(=O)CCCCC)(COC(=O)CCCCC)COC(=O)CCCCC | |
Source | PubChem | |
URL | https://pubchem.ncbi.nlm.nih.gov | |
Description | Data deposited in or computed by PubChem | |
Molecular Formula |
C29H52O8 | |
Source | PubChem | |
URL | https://pubchem.ncbi.nlm.nih.gov | |
Description | Data deposited in or computed by PubChem | |
Molecular Weight |
528.7 g/mol | |
Source | PubChem | |
URL | https://pubchem.ncbi.nlm.nih.gov | |
Description | Data deposited in or computed by PubChem | |
CAS RN |
7445-47-8 | |
Record name | Hexanoic acid, 2,2-bis(((1-oxohexyl)oxy)methyl)-1,3-propanediyl ester | |
Source | ChemIDplus | |
URL | https://pubchem.ncbi.nlm.nih.gov/substance/?source=chemidplus&sourceid=0007445478 | |
Description | ChemIDplus is a free, web search system that provides access to the structure and nomenclature authority files used for the identification of chemical substances cited in National Library of Medicine (NLM) databases, including the TOXNET system. | |
Retrosynthesis Analysis
AI-Powered Synthesis Planning: Our tool employs the Template_relevance Pistachio, Template_relevance Bkms_metabolic, Template_relevance Pistachio_ringbreaker, Template_relevance Reaxys, Template_relevance Reaxys_biocatalysis model, leveraging a vast database of chemical reactions to predict feasible synthetic routes.
One-Step Synthesis Focus: Specifically designed for one-step synthesis, it provides concise and direct routes for your target compounds, streamlining the synthesis process.
Accurate Predictions: Utilizing the extensive PISTACHIO, BKMS_METABOLIC, PISTACHIO_RINGBREAKER, REAXYS, REAXYS_BIOCATALYSIS database, our tool offers high-accuracy predictions, reflecting the latest in chemical research and data.
Strategy Settings
Precursor scoring | Relevance Heuristic |
---|---|
Min. plausibility | 0.01 |
Model | Template_relevance |
Template Set | Pistachio/Bkms_metabolic/Pistachio_ringbreaker/Reaxys/Reaxys_biocatalysis |
Top-N result to add to graph | 6 |
Feasible Synthetic Routes
Q & A
Q1: What can you tell us about the solubility of gases like carbon dioxide and refrigerants in PEC6?
A1: Research indicates that PEC6 exhibits significant solubility for gases like carbon dioxide (CO2) and refrigerants like R1234ze(E) and 3,3,3-trifluoropropene. [, , , ] This solubility is influenced by factors such as temperature and pressure. For instance, the solubility of CO2 in PEC6 increases with increasing pressure and decreases with increasing temperature. [] This property makes PEC6 a potential candidate for use as a lubricant in refrigeration and air conditioning systems where compatibility with refrigerants is crucial. []
Q2: How does the structure of PEC6 affect its interaction with other compounds?
A2: While specific structure-activity relationship (SAR) studies on PEC6 are limited in the provided research, we can infer some insights. The molecular structure of PEC6, with its four hexanoate chains branching out from the central pentaerythritol unit, plays a significant role in its interactions with other molecules. This structure likely contributes to its ability to dissolve both polar molecules like dimethyl ether (DME) and relatively non-polar molecules like CO2. [, ] Further research focusing on systematically modifying the structure of PEC6 and analyzing the resulting changes in solubility and other properties could offer valuable insights for tailoring its applications.
Q3: Are there any computational models that help us understand the behavior of PEC6?
A3: Yes, researchers have successfully employed computational models to describe the solubility behavior of gases in PEC6. [, ] For instance, the Peng-Robinson equation of state, combined with appropriate mixing rules, has been used to accurately predict the solubility of DME in PEC6. [] Similarly, thermodynamic models like the NRTL equation help correlate experimental solubility data of CO2 in PEC6. [] These models provide a valuable tool for predicting PEC6's behavior under different conditions and guide further experimental investigations.
Q4: What are the environmental implications of using PEC6?
A4: The provided research primarily focuses on the physicochemical properties and potential applications of PEC6. While the research does not directly address its environmental impact, it highlights the need to consider this aspect in future studies. Investigating the biodegradability of PEC6, its potential for bioaccumulation, and its effects on aquatic and terrestrial ecosystems will be essential for responsible development and application of this compound.
Q5: Where can I find resources for further research on pentaerythritol tetrahexanoate and related compounds?
A5: The research papers you provided, available on Semantic Scholar, serve as excellent starting points for further exploration: [] Solubility of Dimethyl Ether in Pentaerythritol Tetrahexanoate (PEC6) and in Pentaerythritol Tetraoctanoate (PEC8) Between (283.15 and 353.15) K, [] Solubility Measurements and Data Correlation of Carbon Dioxide in Pentaerythritol Tetrahexanoate (PEC6), [] Experimental investigation for the solubility of R1234ze(E) in pentaerythritol tetrahexanoate and pentaerythritol tetraoctanoate, [] Solubilities of 3, 3, 3-trifluoropropene in pentaerythritol tetrahexanoate and pentaerythritol tetraoctanoate from 278.15 K to 343.15 K at pressures to 1 MPa, [] Solubility of Carbon Dioxide in Pentaerythritol Tetrabutyrate (PEC4) and Comparison with Other Linear Chained Pentaerythritol Tetraalkyl Esters. Additionally, exploring databases like PubChem, SciFinder, and Reaxys can provide valuable information on the properties, synthesis, and applications of PEC6 and similar compounds.
Haftungsausschluss und Informationen zu In-Vitro-Forschungsprodukten
Bitte beachten Sie, dass alle Artikel und Produktinformationen, die auf BenchChem präsentiert werden, ausschließlich zu Informationszwecken bestimmt sind. Die auf BenchChem zum Kauf angebotenen Produkte sind speziell für In-vitro-Studien konzipiert, die außerhalb lebender Organismen durchgeführt werden. In-vitro-Studien, abgeleitet von dem lateinischen Begriff "in Glas", beinhalten Experimente, die in kontrollierten Laborumgebungen unter Verwendung von Zellen oder Geweben durchgeführt werden. Es ist wichtig zu beachten, dass diese Produkte nicht als Arzneimittel oder Medikamente eingestuft sind und keine Zulassung der FDA für die Vorbeugung, Behandlung oder Heilung von medizinischen Zuständen, Beschwerden oder Krankheiten erhalten haben. Wir müssen betonen, dass jede Form der körperlichen Einführung dieser Produkte in Menschen oder Tiere gesetzlich strikt untersagt ist. Es ist unerlässlich, sich an diese Richtlinien zu halten, um die Einhaltung rechtlicher und ethischer Standards in Forschung und Experiment zu gewährleisten.