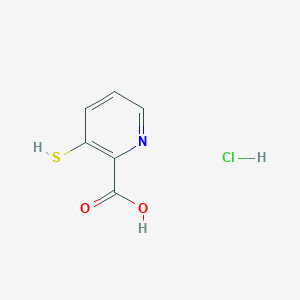
3-Mercaptopicolinic Acid Hydrochloride
Übersicht
Beschreibung
SKF-34288 Hydrochlorid, auch bekannt als 3-Mercaptopicolinsäure-Hydrochlorid, ist ein oral aktiver Inhibitor der Phosphoenolpyruvat-Carboxykinase (PEPCK). Diese Verbindung ist bekannt für ihre potente hypoglykämische Wirkung, die durch die Hemmung der Glucosesynthese erzielt wird. Sie hemmt auch den Asparagin-Stoffwechsel, was zu einer Erhöhung der Aminosäuren und Amide führt .
Herstellungsmethoden
Die Synthese von SKF-34288 Hydrochlorid beinhaltet die Herstellung von 3-Mercaptopicolinsäure, gefolgt von ihrer Umwandlung in das Hydrochloridsalz. Die spezifischen Synthesewege und Reaktionsbedingungen sind urheberrechtlich geschützt und werden in der öffentlichen Literatur nicht allgemein bekannt gegeben. Es ist bekannt, dass die Verbindung unter kontrollierten Laborbedingungen hergestellt wird, um eine hohe Reinheit und Wirksamkeit zu gewährleisten .
Chemische Reaktionsanalyse
SKF-34288 Hydrochlorid unterliegt verschiedenen Arten von chemischen Reaktionen, darunter:
Oxidation: Diese Reaktion kann zur Bildung von Disulfiden führen.
Reduktion: Die Verbindung kann zu ihrem entsprechenden Thiol reduziert werden.
Substitution: Sie kann nucleophile Substitutionsreaktionen eingehen, insbesondere an der Mercaptogruppe.
Häufig verwendete Reagenzien in diesen Reaktionen sind Oxidationsmittel wie Wasserstoffperoxid, Reduktionsmittel wie Natriumborhydrid und Nucleophile wie Alkylhalogenide. Die Hauptprodukte, die aus diesen Reaktionen entstehen, sind Disulfide, Thiole und substituierte Derivate .
Wissenschaftliche Forschungsanwendungen
SKF-34288 Hydrochlorid hat eine breite Palette von Anwendungen in der wissenschaftlichen Forschung:
Chemie: Es wird als Werkzeug zur Untersuchung der Enzyminhibition, insbesondere von PEPCK, verwendet.
Biologie: Die Verbindung wird zur Untersuchung von Stoffwechselwegen verwendet, die die Glucosesynthese und den Aminosäurestoffwechsel betreffen.
Medizin: SKF-34288 Hydrochlorid wird auf seine potenziellen therapeutischen Wirkungen bei der Behandlung von Hyperglykämie und Diabetes untersucht.
Industrie: Es wird bei der Entwicklung neuer Arzneimittel und als Referenzverbindung in biochemischen Assays verwendet
Wirkmechanismus
SKF-34288 Hydrochlorid übt seine Wirkung aus, indem es die Phosphoenolpyruvat-Carboxykinase (PEPCK) im Gluconeogenese-Weg spezifisch hemmt. Diese Hemmung führt zu einer Abnahme der Glucosesynthese, was zu hypoglykämischen Wirkungen führt. Darüber hinaus hemmt die Verbindung den Asparagin-Stoffwechsel, was zu einer Erhöhung der Aminosäuren und Amide führt .
Wirkmechanismus
The inhibition of gluconeogenesis by 3-Mercaptopicolinic Acid Hydrochloride occurs primarily by decreasing the rate of product formation while having relatively minor effects on the apparent Michaelis constants for substrates. It is a noncompetitive inhibitor with respect to both oxaloacetate and MnGTP2-, suggesting that PEPCK is the target of this inhibitor .
Zukünftige Richtungen
Biochemische Analyse
Biochemical Properties
3-Mercaptopicolinic Acid Hydrochloride plays a crucial role in biochemical reactions by inhibiting the enzyme phosphoenolpyruvate carboxykinase (PEPCK). This inhibition leads to a decrease in gluconeogenesis, which is the metabolic pathway that results in the generation of glucose from non-carbohydrate substrates. The compound interacts with PEPCK by binding to its active site, thereby preventing the enzyme from catalyzing the conversion of oxaloacetate to phosphoenolpyruvate . Additionally, this compound has been shown to inhibit asparagine metabolism, leading to an increase in amino acids and amides .
Cellular Effects
This compound has profound effects on various types of cells and cellular processes. In vitro studies have demonstrated that this compound reduces the proliferation of regulatory T cells (T reg cells) and induces myogenic differentiation of C2C12 cells . Furthermore, this compound has been shown to reduce colony growth formation of MCF7 cells, a breast cancer cell line . These effects suggest that the compound influences cell signaling pathways, gene expression, and cellular metabolism.
Molecular Mechanism
The molecular mechanism of action of this compound involves its binding interactions with biomolecules and enzyme inhibition. By binding to the active site of PEPCK, the compound inhibits the enzyme’s activity, leading to a decrease in gluconeogenesis . This inhibition results in lower glucose synthesis, which can have significant implications for metabolic regulation. Additionally, this compound affects gene expression by reducing the mRNA expression of genes related to serine biosynthesis .
Temporal Effects in Laboratory Settings
In laboratory settings, the effects of this compound have been observed to change over time. The compound is stable under standard storage conditions, but its activity may degrade over extended periods. In vitro studies have shown that this compound can inhibit cell proliferation and induce differentiation over a period of 48 hours . In vivo studies have demonstrated that the compound can lower blood glucose levels in starved rats when administered orally .
Dosage Effects in Animal Models
The effects of this compound vary with different dosages in animal models. In starved rats, oral administration of the compound at doses ranging from 37.5 to 150 mg/kg resulted in a dose-dependent reduction in blood glucose levels . Additionally, subcutaneous injection of 25 mg/kg of the compound was able to neutralize salbutamol-mediated hyperglycemia in rabbits . High doses of this compound may lead to toxic or adverse effects, highlighting the importance of dosage optimization in experimental settings.
Metabolic Pathways
This compound is involved in metabolic pathways related to gluconeogenesis and amino acid metabolism. By inhibiting PEPCK, the compound disrupts the conversion of oxaloacetate to phosphoenolpyruvate, thereby reducing glucose synthesis . Additionally, the compound inhibits asparagine metabolism, leading to an increase in amino acids and amides . These effects on metabolic pathways can have significant implications for metabolic flux and metabolite levels.
Transport and Distribution
The transport and distribution of this compound within cells and tissues are influenced by its interactions with transporters and binding proteins. The compound is known to be soluble in water, which facilitates its distribution within biological systems . Specific transporters or binding proteins involved in its cellular uptake and distribution have not been extensively characterized.
Vorbereitungsmethoden
The synthesis of SKF-34288 hydrochloride involves the preparation of 3-Mercaptopicolinic acid, followed by its conversion to the hydrochloride salt. The specific synthetic routes and reaction conditions are proprietary and not widely disclosed in public literature. it is known that the compound is prepared under controlled laboratory conditions to ensure high purity and efficacy .
Analyse Chemischer Reaktionen
SKF-34288 hydrochloride undergoes several types of chemical reactions, including:
Oxidation: This reaction can lead to the formation of disulfides.
Reduction: The compound can be reduced to its corresponding thiol.
Substitution: It can undergo nucleophilic substitution reactions, particularly at the mercapto group.
Common reagents used in these reactions include oxidizing agents like hydrogen peroxide, reducing agents like sodium borohydride, and nucleophiles like alkyl halides. The major products formed from these reactions include disulfides, thiols, and substituted derivatives .
Vergleich Mit ähnlichen Verbindungen
SKF-34288 Hydrochlorid ist einzigartig aufgrund seiner spezifischen Hemmung von PEPCK und seiner potenten hypoglykämischen Wirkungen. Zu ähnlichen Verbindungen gehören:
3-Mercaptopicolinsäure: Die Stammverbindung ohne das Hydrochloridsalz.
Phenylhydrazin-Derivate: Bekannt für ihre enzymhemmenden Eigenschaften.
Thiosemicarbazone: Verbindungen mit ähnlichen Stoffwechseleffekten.
Diese Verbindungen weisen einige strukturelle Ähnlichkeiten auf, unterscheiden sich jedoch in ihren spezifischen Zielmolekülen und ihrer Gesamtwirksamkeit .
Eigenschaften
IUPAC Name |
3-sulfanylpyridine-2-carboxylic acid;hydrochloride | |
---|---|---|
Source | PubChem | |
URL | https://pubchem.ncbi.nlm.nih.gov | |
Description | Data deposited in or computed by PubChem | |
InChI |
InChI=1S/C6H5NO2S.ClH/c8-6(9)5-4(10)2-1-3-7-5;/h1-3,10H,(H,8,9);1H | |
Source | PubChem | |
URL | https://pubchem.ncbi.nlm.nih.gov | |
Description | Data deposited in or computed by PubChem | |
InChI Key |
DYGYEUUVNLEHJP-UHFFFAOYSA-N | |
Source | PubChem | |
URL | https://pubchem.ncbi.nlm.nih.gov | |
Description | Data deposited in or computed by PubChem | |
Canonical SMILES |
C1=CC(=C(N=C1)C(=O)O)S.Cl | |
Source | PubChem | |
URL | https://pubchem.ncbi.nlm.nih.gov | |
Description | Data deposited in or computed by PubChem | |
Molecular Formula |
C6H6ClNO2S | |
Source | PubChem | |
URL | https://pubchem.ncbi.nlm.nih.gov | |
Description | Data deposited in or computed by PubChem | |
DSSTOX Substance ID |
DTXSID90641042 | |
Record name | 3-Sulfanylpyridine-2-carboxylic acid--hydrogen chloride (1/1) | |
Source | EPA DSSTox | |
URL | https://comptox.epa.gov/dashboard/DTXSID90641042 | |
Description | DSSTox provides a high quality public chemistry resource for supporting improved predictive toxicology. | |
Molecular Weight |
191.64 g/mol | |
Source | PubChem | |
URL | https://pubchem.ncbi.nlm.nih.gov | |
Description | Data deposited in or computed by PubChem | |
CAS No. |
320386-54-7 | |
Record name | 3-Sulfanylpyridine-2-carboxylic acid--hydrogen chloride (1/1) | |
Source | EPA DSSTox | |
URL | https://comptox.epa.gov/dashboard/DTXSID90641042 | |
Description | DSSTox provides a high quality public chemistry resource for supporting improved predictive toxicology. | |
Retrosynthesis Analysis
AI-Powered Synthesis Planning: Our tool employs the Template_relevance Pistachio, Template_relevance Bkms_metabolic, Template_relevance Pistachio_ringbreaker, Template_relevance Reaxys, Template_relevance Reaxys_biocatalysis model, leveraging a vast database of chemical reactions to predict feasible synthetic routes.
One-Step Synthesis Focus: Specifically designed for one-step synthesis, it provides concise and direct routes for your target compounds, streamlining the synthesis process.
Accurate Predictions: Utilizing the extensive PISTACHIO, BKMS_METABOLIC, PISTACHIO_RINGBREAKER, REAXYS, REAXYS_BIOCATALYSIS database, our tool offers high-accuracy predictions, reflecting the latest in chemical research and data.
Strategy Settings
Precursor scoring | Relevance Heuristic |
---|---|
Min. plausibility | 0.01 |
Model | Template_relevance |
Template Set | Pistachio/Bkms_metabolic/Pistachio_ringbreaker/Reaxys/Reaxys_biocatalysis |
Top-N result to add to graph | 6 |
Feasible Synthetic Routes
Q1: How does 3-Mercaptopicolinic Acid Hydrochloride interact with Pck1 and affect acute pancreatitis according to the research?
A1: The research paper primarily focuses on the in vivo effects of 3-MPA on acute pancreatitis, highlighting its therapeutic potential. While it identifies Pck1 as a target, it doesn't delve into the specific molecular mechanism of 3-MPA's interaction with this enzyme. The study demonstrates that administering 3-MPA effectively inhibits Pck1 activity in the intestinal epithelial cells of mice with acute pancreatitis []. This inhibition leads to a cascade of beneficial effects, including:
- Improved Intestinal Barrier Function: 3-MPA reduces intestinal permeability, likely by increasing the expression of tight junction proteins and decreasing epithelial cell death [].
- Modulated Immune Response: Treatment with 3-MPA helps restore a balanced intestinal immune response, characterized by an increased ratio of M2 to M1 macrophages and higher secretory immunoglobulin A (sIgA) levels, alongside reduced neutrophil infiltration [].
- Alleviated Pancreatic and Intestinal Injury: The study observes reduced pancreatic and intestinal damage in mice treated with 3-MPA, evidenced by improved histopathological scores [].
Haftungsausschluss und Informationen zu In-Vitro-Forschungsprodukten
Bitte beachten Sie, dass alle Artikel und Produktinformationen, die auf BenchChem präsentiert werden, ausschließlich zu Informationszwecken bestimmt sind. Die auf BenchChem zum Kauf angebotenen Produkte sind speziell für In-vitro-Studien konzipiert, die außerhalb lebender Organismen durchgeführt werden. In-vitro-Studien, abgeleitet von dem lateinischen Begriff "in Glas", beinhalten Experimente, die in kontrollierten Laborumgebungen unter Verwendung von Zellen oder Geweben durchgeführt werden. Es ist wichtig zu beachten, dass diese Produkte nicht als Arzneimittel oder Medikamente eingestuft sind und keine Zulassung der FDA für die Vorbeugung, Behandlung oder Heilung von medizinischen Zuständen, Beschwerden oder Krankheiten erhalten haben. Wir müssen betonen, dass jede Form der körperlichen Einführung dieser Produkte in Menschen oder Tiere gesetzlich strikt untersagt ist. Es ist unerlässlich, sich an diese Richtlinien zu halten, um die Einhaltung rechtlicher und ethischer Standards in Forschung und Experiment zu gewährleisten.