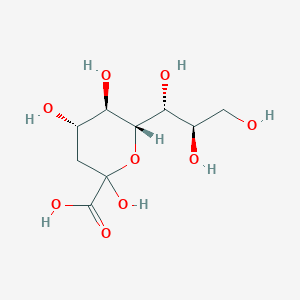
2-Keto-3-Desoxy-Nononsäure
Übersicht
Beschreibung
3-Desoxy-D-glycero-D-galacto-2-nonuloson Säure ist eine deaminierte Sialinsäure, eine Art Zuckersäure, die eine entscheidende Rolle in verschiedenen biologischen Prozessen spielt. Sie wurde erstmals in den Glykoproteinen von Regenbogenforelleneiern identifiziert und ist bekannt für ihr Vorkommen an den nichtreduzierenden Enden von Oligosialylketten . Diese Verbindung ist bedeutsam für Zellfunktionen wie Zellhaftung, Signalerkennung und Tumorprogression .
Wissenschaftliche Forschungsanwendungen
3-Desoxy-D-glycero-D-galacto-2-nonuloson Säure hat eine breite Palette von Anwendungen in der wissenschaftlichen Forschung:
Chemie: Sie wird als Vorläufer für die Synthese anderer Sialinsäuren und verwandter Verbindungen verwendet.
Wirkmechanismus
Der Mechanismus, durch den 3-Desoxy-D-glycero-D-galacto-2-nonuloson Säure ihre Wirkung entfaltet, beinhaltet ihre Wechselwirkung mit spezifischen molekularen Zielstrukturen und Signalwegen. Es ist bekannt, dass sie an Zelloberflächenrezeptoren bindet und so die Zellhaftung und Signalwege beeinflusst. Diese Wechselwirkung kann Immunreaktionen modulieren und das Verhalten von Krebszellen beeinflussen, was sie zu einem Ziel für therapeutische Interventionen macht .
Wirkmechanismus
Target of Action
2-Keto-3-deoxy-nononic acid, also known as 3-Deoxy-D-Glycero-D-Galacto-Non-2-ulopyranosonic Acid or KDN, is a member of the sialic acid family . It is found in almost all types of glycoconjugates, including glycolipids, glycoproteins, and capsular polysaccharides . KDN residues are linked to almost all glycan structures in place of Neu5Ac .
Mode of Action
KDN interacts with its targets through its residues, which are linked to almost all glycan structures . All linkage types known for Neu5Ac; α2,3-, α2,4-, α2,6-, and α2,8- are also found for KDN .
Biochemical Pathways
KDN is biosynthesized de novo using mannose as a precursor sugar, which is activated to CMP-KDN and transferred to acceptor sugar residues . These reactions are catalyzed by enzymes, some of which preferably recognize KDN, but many others prefer Neu5Ac to KDN . The pathway consists of the following three sequential reactions :
Pharmacokinetics
It is known that kdn is biosynthesized de novo in the body .
Result of Action
KDN plays a significant role in cellular recognition and protein-carbohydrate interactions . It is also known to influence the biological properties of a cell . Elevated expression of KDN was found in fetal human red blood cells compared with adult red blood cells, and ovarian tumor tissues compared with normal controls .
Action Environment
The action of KDN can be influenced by various environmental factors. For instance, in human neuroblastoma, nephroblastoma, and breast cancer, short-chain KDN through α2,8 linkages forms polysialic acid, which can weaken cell-cell adhesion and interactions between cells and the matrix, thereby promoting cancer cell separation, infiltration, and distant metastasis .
Biochemische Analyse
Biochemical Properties
2-Keto-3-Deoxy-Nononic Acid is found in almost all types of glycoconjugates, including glycolipids, glycoproteins, and capsular polysaccharides . It interacts with various enzymes, some of which preferably recognize 2-Keto-3-Deoxy-Nononic Acid, but many others prefer N-acetylneuraminic acid to 2-Keto-3-Deoxy-Nononic Acid .
Cellular Effects
Elevated expression of 2-Keto-3-Deoxy-Nononic Acid was found in fetal human red blood cells compared with adult red blood cells, and ovarian tumor tissues compared with normal controls . It influences cell function by modulating the biological properties of a cell .
Molecular Mechanism
The mechanism of action of 2-Keto-3-Deoxy-Nononic Acid involves its biosynthesis de novo using mannose as a precursor sugar, which is activated to CMP-2-Keto-3-Deoxy-Nononic Acid and transferred to acceptor sugar residues .
Metabolic Pathways
2-Keto-3-Deoxy-Nononic Acid is involved in the amino sugar and nucleotide sugar metabolism pathway . It interacts with enzymes and cofactors in this pathway, potentially affecting metabolic flux or metabolite levels .
Transport and Distribution
Given its presence in various types of glycoconjugates, it’s likely that it interacts with transporters or binding proteins, influencing its localization or accumulation .
Vorbereitungsmethoden
Synthesewege und Reaktionsbedingungen
Die Synthese von 3-Desoxy-D-glycero-D-galacto-2-nonuloson Säure kann durch einen mehrstufigen Prozess erfolgen. Eine bemerkenswerte Methode beinhaltet die Umwandlung von enantiomerenreinem cis-1,2-Dihydrobrenzcatechin, das durch mikrobielle Oxidation von Chlorbenzol erhalten wird, in die Zielverbindung über eine zehnstufige Reaktionssequenz . Dieser Prozess umfasst mehrere Schlüsselschritte wie Oxidation, Reduktion und Schutz-Entschützungskreisläufe, um die gewünschte Stereochemie und funktionellen Gruppen zu erreichen.
Industrielle Produktionsverfahren
Industrielle Produktionsverfahren für 3-Desoxy-D-glycero-D-galacto-2-nonuloson Säure sind weniger dokumentiert, aber sie beinhalten wahrscheinlich ähnliche Synthesewege mit Optimierungen für die Hochskalierung. Diese Optimierungen können die Verwendung effizienterer Katalysatoren, verbesserte Reaktionsbedingungen und optimierte Reinigungsprozesse umfassen, um die Ausbeute zu verbessern und die Kosten zu senken.
Analyse Chemischer Reaktionen
Arten von Reaktionen
3-Desoxy-D-glycero-D-galacto-2-nonuloson Säure durchläuft verschiedene chemische Reaktionen, darunter:
Oxidation: Diese Reaktion kann die in dem Molekül vorhandenen Hydroxylgruppen modifizieren.
Reduktion: Reduktionsreaktionen können die Carbonylgruppen anvisieren, um Alkohole zu bilden.
Substitution: Funktionelle Gruppen im Molekül können unter geeigneten Bedingungen durch andere Gruppen substituiert werden.
Häufige Reagenzien und Bedingungen
Häufig verwendete Reagenzien in diesen Reaktionen umfassen Oxidationsmittel wie Kaliumpermanganat, Reduktionsmittel wie Natriumborhydrid und verschiedene Säuren und Basen für Substitutionsreaktionen. Die Bedingungen umfassen typischerweise kontrollierte Temperaturen und pH-Werte, um den gewünschten Reaktionsweg und die Produktbildung sicherzustellen.
Hauptsächlich gebildete Produkte
Die hauptsächlich aus diesen Reaktionen gebildeten Produkte hängen von den spezifischen Bedingungen und Reagenzien ab. Zum Beispiel kann die Oxidation zur Bildung von Carbonsäuren führen, während die Reduktion Alkoholderivate erzeugen kann.
Vergleich Mit ähnlichen Verbindungen
3-Desoxy-D-glycero-D-galacto-2-nonuloson Säure ist unter den Sialinsäuren aufgrund ihrer deaminierten Struktur einzigartig. Zu ähnlichen Verbindungen gehören:
N-Acetylneuraminsäure: Die häufigste Sialinsäure, die an vielen biologischen Prozessen beteiligt ist.
N-Glycolylneuraminsäure: Eine weitere Variante der Sialinsäure mit einer Hydroxylgruppe anstelle der Acetylgruppe.
2-Keto-3-desoxy-D-glycero-D-galacto-nononsäure: Eine strukturell ähnliche Verbindung mit unterschiedlichen funktionellen Gruppen.
Eigenschaften
IUPAC Name |
(4S,5R,6R)-2,4,5-trihydroxy-6-[(1R,2R)-1,2,3-trihydroxypropyl]oxane-2-carboxylic acid | |
---|---|---|
Source | PubChem | |
URL | https://pubchem.ncbi.nlm.nih.gov | |
Description | Data deposited in or computed by PubChem | |
InChI |
InChI=1S/C9H16O9/c10-2-4(12)6(14)7-5(13)3(11)1-9(17,18-7)8(15)16/h3-7,10-14,17H,1-2H2,(H,15,16)/t3-,4+,5+,6+,7+,9?/m0/s1 | |
Source | PubChem | |
URL | https://pubchem.ncbi.nlm.nih.gov | |
Description | Data deposited in or computed by PubChem | |
InChI Key |
CLRLHXKNIYJWAW-QBTAGHCHSA-N | |
Source | PubChem | |
URL | https://pubchem.ncbi.nlm.nih.gov | |
Description | Data deposited in or computed by PubChem | |
Canonical SMILES |
C1C(C(C(OC1(C(=O)O)O)C(C(CO)O)O)O)O | |
Source | PubChem | |
URL | https://pubchem.ncbi.nlm.nih.gov | |
Description | Data deposited in or computed by PubChem | |
Isomeric SMILES |
C1[C@@H]([C@H]([C@@H](OC1(C(=O)O)O)[C@@H]([C@@H](CO)O)O)O)O | |
Source | PubChem | |
URL | https://pubchem.ncbi.nlm.nih.gov | |
Description | Data deposited in or computed by PubChem | |
Molecular Formula |
C9H16O9 | |
Source | PubChem | |
URL | https://pubchem.ncbi.nlm.nih.gov | |
Description | Data deposited in or computed by PubChem | |
DSSTOX Substance ID |
DTXSID50661882 | |
Record name | 3-Deoxy-D-glycero-D-galacto-2-nonulopyranosonic acid | |
Source | EPA DSSTox | |
URL | https://comptox.epa.gov/dashboard/DTXSID50661882 | |
Description | DSSTox provides a high quality public chemistry resource for supporting improved predictive toxicology. | |
Molecular Weight |
268.22 g/mol | |
Source | PubChem | |
URL | https://pubchem.ncbi.nlm.nih.gov | |
Description | Data deposited in or computed by PubChem | |
CAS No. |
153666-19-4 | |
Record name | 3-Deoxy-D-glycero-D-galacto-2-nonulopyranosonic acid | |
Source | EPA DSSTox | |
URL | https://comptox.epa.gov/dashboard/DTXSID50661882 | |
Description | DSSTox provides a high quality public chemistry resource for supporting improved predictive toxicology. | |
Retrosynthesis Analysis
AI-Powered Synthesis Planning: Our tool employs the Template_relevance Pistachio, Template_relevance Bkms_metabolic, Template_relevance Pistachio_ringbreaker, Template_relevance Reaxys, Template_relevance Reaxys_biocatalysis model, leveraging a vast database of chemical reactions to predict feasible synthetic routes.
One-Step Synthesis Focus: Specifically designed for one-step synthesis, it provides concise and direct routes for your target compounds, streamlining the synthesis process.
Accurate Predictions: Utilizing the extensive PISTACHIO, BKMS_METABOLIC, PISTACHIO_RINGBREAKER, REAXYS, REAXYS_BIOCATALYSIS database, our tool offers high-accuracy predictions, reflecting the latest in chemical research and data.
Strategy Settings
Precursor scoring | Relevance Heuristic |
---|---|
Min. plausibility | 0.01 |
Model | Template_relevance |
Template Set | Pistachio/Bkms_metabolic/Pistachio_ringbreaker/Reaxys/Reaxys_biocatalysis |
Top-N result to add to graph | 6 |
Feasible Synthetic Routes
Q1: What is the molecular formula and weight of KDN?
A1: The molecular formula of KDN is C9H16O10, and its molecular weight is 268.22 g/mol.
Q2: What spectroscopic data is available for characterizing KDN?
A2: Researchers commonly utilize techniques like 1H-NMR, 13C-NMR, and circular dichroism (CD) spectral analysis to determine the structure and stereochemistry of KDN and its derivatives. [, , ]
Q3: Are there different methods to synthesize KDN?
A3: Yes, KDN can be synthesized through various methods:
- Base-catalyzed condensation: This approach efficiently yields KDN. []
- Condensation of oxalacetic acid with D-mannose: This method is followed by several steps to obtain the desired KDN derivative. []
- Horner-Wittig reactions: This pathway, utilizing specific starting materials, leads to the synthesis of KDN derivatives. []
- Desymmetrization by ring-closing metathesis: This method enables the introduction of oxygen functionality in a stereocontrolled manner. []
Q4: Can KDN be synthesized from a non-carbohydrate source?
A4: Yes, the first total synthesis of (+)-KDN was achieved using enantiopure cis-1,2-dihydrocatechol, a product obtained by microbial oxidation of chlorobenzene. [, ]
Q5: Where is KDN found in nature?
A5: KDN is found in a variety of organisms, including:
- Fish: Notably, the liver of the loach (Misgurnus fossilis) contains a sialidase enzyme (KDNase) that specifically cleaves KDN. [, ]
- Bacteria: Certain plant pathogenic Streptomyces species incorporate KDN into their cell wall polymers. [, ]
- Mammals: KDN has been detected in various rat organs and tissues, with notable age-related variations observed in the liver. [, ]
- Microalgae: The haptophyte Prymnesium parvum has been found to possess a KDN biosynthesis pathway. []
Q6: What are the biological roles of KDN?
A6: While research on KDN function is ongoing, some insights include:
- Cell wall component: In plant pathogenic Streptomyces, KDN-containing polymers may contribute to the attachment of these bacteria to host plant cells. []
- Potential role in mammals: Studies suggest that KDN biosynthesis might help regulate physiological mannose levels in mammals. Additionally, under specific conditions, KDN-containing glycans could elicit immune responses. []
Q7: What are some important derivatives and analogs of KDN?
A7: Several derivatives and analogs of KDN have been synthesized, including:
- Aryl-α-glycosides: These derivatives have been synthesized and characterized using NMR and CD spectral analysis. []
- Nucleoside analogues: Mono- and disaccharide nucleoside analogues have been synthesized using various reaction conditions, their stereochemistry investigated using NMR. [, ]
- α-N-Glycosides with nucleobases: These derivatives, synthesized using uracil, thymine, and other nucleobases, have been studied for their photocycloaddition properties. [, ]
- Phosphonic acid analogues: These synthetic analogs have shown moderate activity as potential sialidase inhibitors. []
- 4-acylamino-2,6-anhydro-2,3,4-trideoxy-D-glycero-D-galacto-non-2-enoic acids: These compounds, synthesized from KDN, have been structurally characterized using techniques like NMR and X-ray crystallography. [, ]
- Ganglioside GM4 and GM3 analogs: These synthetic analogs, containing KDN in place of N-acetylneuraminic acid, have been successfully produced. []
- Tetrazolyl derivatives: These have been explored as useful glycosyl donors for synthesizing O- and C-glycosides. []
Q8: How does the structure of KDN impact its activity?
A8: While specific structure-activity relationships require further investigation, some modifications have demonstrated effects:
- Gangliosides: Studies on chemically synthesized GM3 and GM4, containing KDN, showed that the length of the fatty acyl chain inversely correlates with immunosuppressive activity. Modifications like hydroxylation of the fatty acyl group can decrease immunosuppressive activity. []
- Sialidase inhibition: Replacing the carboxyl group of KDN with a phosphonic acid group resulted in moderate sialidase inhibitory activity. []
Q9: How is KDN typically analyzed and quantified?
A9: Several analytical methods are employed for KDN:
- High-performance liquid chromatography (HPLC): This technique, particularly with a strongly basic anion-exchange resin, is used to analyze and isolate KDN. []
- HPLC with pulsed amperometric detection (PAD): This method allows simultaneous analysis of KDN alongside other sialic acids like Neu5Ac and Neu5Gc. []
- Gas chromatography/mass spectrometry (GC/MS): This technique is valuable for characterizing KDN and other sialic acid-like monosaccharides. The method involves converting KDN to its acetylated lactone form prior to analysis. []
Q10: Does KDN have any specific applications?
A10: Research on KDN applications is ongoing, but some potential areas include:
- Glycoscience research: KDN and its derivatives are valuable tools for studying sialic acid biology and enzymology. [, ]
- Molecular probes: Synthetic KDN derivatives can be used to investigate the substrate specificity of enzymes like human α1,3-fucosyltransferases. []
- Potential therapeutic targets: While still under investigation, KDN-related pathways could be explored for potential therapeutic interventions, particularly in cases where KDN levels are elevated. []
Haftungsausschluss und Informationen zu In-Vitro-Forschungsprodukten
Bitte beachten Sie, dass alle Artikel und Produktinformationen, die auf BenchChem präsentiert werden, ausschließlich zu Informationszwecken bestimmt sind. Die auf BenchChem zum Kauf angebotenen Produkte sind speziell für In-vitro-Studien konzipiert, die außerhalb lebender Organismen durchgeführt werden. In-vitro-Studien, abgeleitet von dem lateinischen Begriff "in Glas", beinhalten Experimente, die in kontrollierten Laborumgebungen unter Verwendung von Zellen oder Geweben durchgeführt werden. Es ist wichtig zu beachten, dass diese Produkte nicht als Arzneimittel oder Medikamente eingestuft sind und keine Zulassung der FDA für die Vorbeugung, Behandlung oder Heilung von medizinischen Zuständen, Beschwerden oder Krankheiten erhalten haben. Wir müssen betonen, dass jede Form der körperlichen Einführung dieser Produkte in Menschen oder Tiere gesetzlich strikt untersagt ist. Es ist unerlässlich, sich an diese Richtlinien zu halten, um die Einhaltung rechtlicher und ethischer Standards in Forschung und Experiment zu gewährleisten.