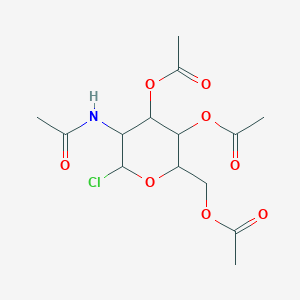
2-Acetamido-3,4,6-tri-O-acetyl-2-deoxy-alpha-D-glucopyranosyl chloride
Übersicht
Beschreibung
2-Acetamido-3,4,6-tri-O-acetyl-2-deoxy-alpha-D-glucopyranosyl chloride (2A3,4,6TAC2DAGC) is a synthetic compound used in a variety of scientific and medical research applications. It is a highly versatile compound that can be used to develop new drugs, investigate biochemical and physiological processes, and conduct laboratory experiments.
Wissenschaftliche Forschungsanwendungen
Antibacterial and Antitubercular Activities
The compound has been used in the synthesis of triethylammonium 2-acetamido-3,4,6-tri-O-acetyl-2-deoxy-D-glucopyranosyl decyl phosphate, which has shown promising antitubercular and antibacterial activities .
Synthesis of Glycosides
The compound is used in the synthesis of glycosides, which are important bioactive molecules with wide distribution in nature. They play critical roles in many biological processes and strongly influence human health .
Enzyme Testing
The compound is used as a substrate for testing hydrolytic enzymes. Synthetic glycosides with chromogenic or fluorogenic groups are often used as substrates for this purpose .
Biomedical Applications
The compound has potential biomedical applications, including wound healing, bone regeneration, antibacterial effect, and oral hygiene. It also discusses the role of chitosan oligosaccharide as a drug carrier for molecular therapies, such as the drug and the gene delivery systems and the role in imaging for tumor and cancer detection .
Immune Response
The compound is used in the study of human lysozyme, a natural non-specific immune protein that participates in the immune response of infants against bacterial and viral infections .
Antimicrobial Activity
The compound is used in the study of Escherichia coli’s ability to grow on a series of acetylated and glycosylated compounds. It is surmised that E. coli maintains low levels of nonspecific esterase activity .
Drug Development
The compound is a key compound in biomedicine used for the synthesis of complex carbohydrate-based drugs. This versatile reagent is utilized in the development of novel pharmaceuticals targeting various diseases, including bacterial and viral infections, cancer, and inflammatory disorders .
Regenerative Medicine
The compound and its derivatives have potential use in regenerative medicine. They have been widely used in food, cosmetics, and pharmaceutical industries and are currently produced by acid hydrolysis of chitin .
Eigenschaften
IUPAC Name |
[(2R,3S,4R,5R,6R)-5-acetamido-3,4-diacetyloxy-6-chlorooxan-2-yl]methyl acetate | |
---|---|---|
Source | PubChem | |
URL | https://pubchem.ncbi.nlm.nih.gov | |
Description | Data deposited in or computed by PubChem | |
InChI |
InChI=1S/C14H20ClNO8/c1-6(17)16-11-13(23-9(4)20)12(22-8(3)19)10(24-14(11)15)5-21-7(2)18/h10-14H,5H2,1-4H3,(H,16,17)/t10-,11-,12-,13-,14+/m1/s1 | |
Source | PubChem | |
URL | https://pubchem.ncbi.nlm.nih.gov | |
Description | Data deposited in or computed by PubChem | |
InChI Key |
NAYYKQAWUWXLPD-KSTCHIGDSA-N | |
Source | PubChem | |
URL | https://pubchem.ncbi.nlm.nih.gov | |
Description | Data deposited in or computed by PubChem | |
Canonical SMILES |
CC(=O)NC1C(C(C(OC1Cl)COC(=O)C)OC(=O)C)OC(=O)C | |
Source | PubChem | |
URL | https://pubchem.ncbi.nlm.nih.gov | |
Description | Data deposited in or computed by PubChem | |
Isomeric SMILES |
CC(=O)N[C@@H]1[C@H]([C@@H]([C@H](O[C@@H]1Cl)COC(=O)C)OC(=O)C)OC(=O)C | |
Source | PubChem | |
URL | https://pubchem.ncbi.nlm.nih.gov | |
Description | Data deposited in or computed by PubChem | |
Molecular Formula |
C14H20ClNO8 | |
Source | PubChem | |
URL | https://pubchem.ncbi.nlm.nih.gov | |
Description | Data deposited in or computed by PubChem | |
Molecular Weight |
365.76 g/mol | |
Source | PubChem | |
URL | https://pubchem.ncbi.nlm.nih.gov | |
Description | Data deposited in or computed by PubChem | |
Product Name |
2-Acetamido-3,4,6-tri-O-acetyl-2-deoxy-alpha-D-glucopyranosyl chloride | |
CAS RN |
3068-34-6 | |
Record name | 2-Acetamido-3,4,6-tri-O-acetyl-2-deoxy-α-D-glucopyranosyl chloride | |
Source | CAS Common Chemistry | |
URL | https://commonchemistry.cas.org/detail?cas_rn=3068-34-6 | |
Description | CAS Common Chemistry is an open community resource for accessing chemical information. Nearly 500,000 chemical substances from CAS REGISTRY cover areas of community interest, including common and frequently regulated chemicals, and those relevant to high school and undergraduate chemistry classes. This chemical information, curated by our expert scientists, is provided in alignment with our mission as a division of the American Chemical Society. | |
Explanation | The data from CAS Common Chemistry is provided under a CC-BY-NC 4.0 license, unless otherwise stated. | |
Record name | 2-acetamido-3,4,6-tri-O-acetyl-2-deoxy-α-D-glucopyranosyl chloride | |
Source | European Chemicals Agency (ECHA) | |
URL | https://echa.europa.eu/substance-information/-/substanceinfo/100.019.387 | |
Description | The European Chemicals Agency (ECHA) is an agency of the European Union which is the driving force among regulatory authorities in implementing the EU's groundbreaking chemicals legislation for the benefit of human health and the environment as well as for innovation and competitiveness. | |
Explanation | Use of the information, documents and data from the ECHA website is subject to the terms and conditions of this Legal Notice, and subject to other binding limitations provided for under applicable law, the information, documents and data made available on the ECHA website may be reproduced, distributed and/or used, totally or in part, for non-commercial purposes provided that ECHA is acknowledged as the source: "Source: European Chemicals Agency, http://echa.europa.eu/". Such acknowledgement must be included in each copy of the material. ECHA permits and encourages organisations and individuals to create links to the ECHA website under the following cumulative conditions: Links can only be made to webpages that provide a link to the Legal Notice page. | |
Retrosynthesis Analysis
AI-Powered Synthesis Planning: Our tool employs the Template_relevance Pistachio, Template_relevance Bkms_metabolic, Template_relevance Pistachio_ringbreaker, Template_relevance Reaxys, Template_relevance Reaxys_biocatalysis model, leveraging a vast database of chemical reactions to predict feasible synthetic routes.
One-Step Synthesis Focus: Specifically designed for one-step synthesis, it provides concise and direct routes for your target compounds, streamlining the synthesis process.
Accurate Predictions: Utilizing the extensive PISTACHIO, BKMS_METABOLIC, PISTACHIO_RINGBREAKER, REAXYS, REAXYS_BIOCATALYSIS database, our tool offers high-accuracy predictions, reflecting the latest in chemical research and data.
Strategy Settings
Precursor scoring | Relevance Heuristic |
---|---|
Min. plausibility | 0.01 |
Model | Template_relevance |
Template Set | Pistachio/Bkms_metabolic/Pistachio_ringbreaker/Reaxys/Reaxys_biocatalysis |
Top-N result to add to graph | 6 |
Feasible Synthetic Routes
Q & A
Q1: What is the significance of 2-Acetamido-3,4,6-tri-O-acetyl-2-deoxy-alpha-D-glucopyranosyl chloride in carbohydrate chemistry?
A1: 2-Acetamido-3,4,6-tri-O-acetyl-2-deoxy-alpha-D-glucopyranosyl chloride serves as a crucial building block in carbohydrate chemistry. It's frequently employed in synthesizing various complex carbohydrates, especially those containing the N-acetyl-D-glucosamine unit. []
Q2: The research paper mentions a "highly concise preparation" of O-deacetylated arylthioglycosides using this compound. Can you elaborate on its role in this process?
A2: The paper [] details a method where 2-Acetamido-3,4,6-tri-O-acetyl-2-deoxy-alpha-D-glucopyranosyl chloride directly reacts with aryl thiols or disulfides. This reaction results in the formation of arylthioglycosides, specifically O-deacetylated derivatives. This approach streamlines the synthesis by circumventing the need for separate deacetylation steps, making it more efficient and concise.
Haftungsausschluss und Informationen zu In-Vitro-Forschungsprodukten
Bitte beachten Sie, dass alle Artikel und Produktinformationen, die auf BenchChem präsentiert werden, ausschließlich zu Informationszwecken bestimmt sind. Die auf BenchChem zum Kauf angebotenen Produkte sind speziell für In-vitro-Studien konzipiert, die außerhalb lebender Organismen durchgeführt werden. In-vitro-Studien, abgeleitet von dem lateinischen Begriff "in Glas", beinhalten Experimente, die in kontrollierten Laborumgebungen unter Verwendung von Zellen oder Geweben durchgeführt werden. Es ist wichtig zu beachten, dass diese Produkte nicht als Arzneimittel oder Medikamente eingestuft sind und keine Zulassung der FDA für die Vorbeugung, Behandlung oder Heilung von medizinischen Zuständen, Beschwerden oder Krankheiten erhalten haben. Wir müssen betonen, dass jede Form der körperlichen Einführung dieser Produkte in Menschen oder Tiere gesetzlich strikt untersagt ist. Es ist unerlässlich, sich an diese Richtlinien zu halten, um die Einhaltung rechtlicher und ethischer Standards in Forschung und Experiment zu gewährleisten.