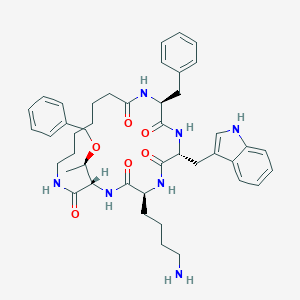
Cyclosomatostatin
Übersicht
Beschreibung
Synthesis Analysis
The synthesis of Cyclosomatostatin involves assembling its amino acid sequence. It is a cyclic peptide composed of the following sequence: Cyclo-(7-aminoheptanoyl-Phe-D-Trp-Lys-Thr) . The modification of amino acids in this sequence contributes to its biological activity.
Molecular Structure Analysis
Cyclosomatostatin has a molecular weight of 779.98 g/mol and the chemical formula C44H57N7O6 . Its structure consists of a cyclic backbone with specific amino acid residues, including phenylalanine (Phe), D-tryptophan (D-Trp), lysine (Lys), and threonine (Thr) .
Physical And Chemical Properties Analysis
Wissenschaftliche Forschungsanwendungen
Neuroscience: Role in Long-Term Potentiation
Cyclosomatostatin, as a somatostatin antagonist, has been found to play a significant role in long-term potentiation at excitatory synapses onto hippocampal somatostatinergic interneurons . It contributes to hippocampus-dependent learning and memory . The application of exogenous somatostatin induces long-term potentiation of excitatory postsynaptic potentials in these interneurons via somatostatin type 1–5 receptors .
Endocrinology: Regulation of Hormone Release
Cyclosomatostatin blocks the effect of somatostatin on the release of growth hormone, insulin, and glucagon . This suggests its potential use in studying the regulation of these hormones and their roles in various physiological processes.
Gastroenterology: Gastric Emptying
Cyclosomatostatin has been found to block the effect of somatostatin on corticotropin-releasing factor (CRF)-induced suppression of gastric emptying . This could be useful in studying gastrointestinal motility disorders.
Neuropharmacology: Modulation of Acetylcholine Release
Cyclosomatostatin can block the effect of somatostatin on the modulation of acetylcholine release . This suggests its potential use in studying neurotransmission and related disorders.
Pharmacology: β-Adrenergic Function
Cyclosomatostatin blocks the effect of somatostatin on β-adrenergic function . This could be useful in studying the role of β-adrenergic receptors in various physiological processes and diseases.
Oncology: Antiproliferative Activity
Cyclosomatostatin may act as a potent antiproliferative agonist in some cell lines . This suggests its potential use in cancer research.
Wirkmechanismus
Target of Action
Cyclosomatostatin is a non-selective somatostatin (sst) receptor antagonist . It primarily targets the somatostatin receptors , which are G protein-coupled receptors . These receptors are normally expressed in the gastrointestinal (GI) tract, pancreas, hypothalamus, and central nervous system (CNS) . They are also expressed in different types of tumors, with the predominant subtype in cancer cells being the ssrt2 subtype .
Mode of Action
Cyclosomatostatin works by blocking the effects of somatostatin on various functions . It inhibits somatostatin receptor type 1 (SSTR1) signaling . It has been reported to act as an sst receptor agonist in human neuroblastoma cell line sh-sy5y .
Biochemical Pathways
Cyclosomatostatin affects several biochemical pathways. It blocks the effect of somatostatin on β-adrenergic function and corticotropin-releasing factor (CRF)-induced suppression of gastric emptying . It also modulates the release of acetylcholine (ACh) and the hormones growth hormone, insulin, and glucagon .
Result of Action
Cyclosomatostatin has various biological activities. It decreases cell proliferation and the size of the ALDH+ cell population, and it inhibits sphere-formation in colorectal cancer (CRC) cells . It also contributes to long-term potentiation at excitatory synapses of somatostatin-expressing interneurons (SOM-INs) by controlling GABA A inhibition .
Action Environment
The action of Cyclosomatostatin can be influenced by various environmental factors. For instance, the presence of endogenous somatostatin can affect the compound’s action . Additionally, the cellular environment, such as the presence of other signaling molecules and the overall state of the cell, can also impact the efficacy and stability of Cyclosomatostatin.
Eigenschaften
IUPAC Name |
(3S,6S,9R,12S)-6-(4-aminobutyl)-12-benzyl-9-(1H-indol-3-ylmethyl)-3-[(1R)-1-phenylmethoxyethyl]-1,4,7,10,13-pentazacycloicosane-2,5,8,11,14-pentone | |
---|---|---|
Source | PubChem | |
URL | https://pubchem.ncbi.nlm.nih.gov | |
Description | Data deposited in or computed by PubChem | |
InChI |
InChI=1S/C44H57N7O6/c1-30(57-29-32-18-8-5-9-19-32)40-44(56)46-25-15-3-2-10-23-39(52)48-37(26-31-16-6-4-7-17-31)42(54)50-38(27-33-28-47-35-21-12-11-20-34(33)35)43(55)49-36(41(53)51-40)22-13-14-24-45/h4-9,11-12,16-21,28,30,36-38,40,47H,2-3,10,13-15,22-27,29,45H2,1H3,(H,46,56)(H,48,52)(H,49,55)(H,50,54)(H,51,53)/t30-,36+,37+,38-,40+/m1/s1 | |
Source | PubChem | |
URL | https://pubchem.ncbi.nlm.nih.gov | |
Description | Data deposited in or computed by PubChem | |
InChI Key |
YHVHQZYJGWGAKN-ZUWUZHNASA-N | |
Source | PubChem | |
URL | https://pubchem.ncbi.nlm.nih.gov | |
Description | Data deposited in or computed by PubChem | |
Canonical SMILES |
CC(C1C(=O)NCCCCCCC(=O)NC(C(=O)NC(C(=O)NC(C(=O)N1)CCCCN)CC2=CNC3=CC=CC=C32)CC4=CC=CC=C4)OCC5=CC=CC=C5 | |
Source | PubChem | |
URL | https://pubchem.ncbi.nlm.nih.gov | |
Description | Data deposited in or computed by PubChem | |
Isomeric SMILES |
C[C@H]([C@H]1C(=O)NCCCCCCC(=O)N[C@H](C(=O)N[C@@H](C(=O)N[C@H](C(=O)N1)CCCCN)CC2=CNC3=CC=CC=C32)CC4=CC=CC=C4)OCC5=CC=CC=C5 | |
Source | PubChem | |
URL | https://pubchem.ncbi.nlm.nih.gov | |
Description | Data deposited in or computed by PubChem | |
Molecular Formula |
C44H57N7O6 | |
Source | PubChem | |
URL | https://pubchem.ncbi.nlm.nih.gov | |
Description | Data deposited in or computed by PubChem | |
DSSTOX Substance ID |
DTXSID901004468 | |
Record name | 6-(4-Aminobutyl)-12-benzyl-3-[1-(benzyloxy)ethyl]-9-[(1H-indol-3-yl)methyl]-1,4,7,10,13-pentaazacycloicosa-1,4,7,10,13-pentaene-2,5,8,11,14-pentol | |
Source | EPA DSSTox | |
URL | https://comptox.epa.gov/dashboard/DTXSID901004468 | |
Description | DSSTox provides a high quality public chemistry resource for supporting improved predictive toxicology. | |
Molecular Weight |
780.0 g/mol | |
Source | PubChem | |
URL | https://pubchem.ncbi.nlm.nih.gov | |
Description | Data deposited in or computed by PubChem | |
Product Name |
Cyclosomatostatin | |
CAS RN |
84211-54-1 | |
Record name | Somatostatin, cyclo-(7-aminoheptanoyl-phenylalanyl-tryptophyl-lysyl-benzylthreonyl)- | |
Source | ChemIDplus | |
URL | https://pubchem.ncbi.nlm.nih.gov/substance/?source=chemidplus&sourceid=0084211541 | |
Description | ChemIDplus is a free, web search system that provides access to the structure and nomenclature authority files used for the identification of chemical substances cited in National Library of Medicine (NLM) databases, including the TOXNET system. | |
Record name | 6-(4-Aminobutyl)-12-benzyl-3-[1-(benzyloxy)ethyl]-9-[(1H-indol-3-yl)methyl]-1,4,7,10,13-pentaazacycloicosa-1,4,7,10,13-pentaene-2,5,8,11,14-pentol | |
Source | EPA DSSTox | |
URL | https://comptox.epa.gov/dashboard/DTXSID901004468 | |
Description | DSSTox provides a high quality public chemistry resource for supporting improved predictive toxicology. | |
Retrosynthesis Analysis
AI-Powered Synthesis Planning: Our tool employs the Template_relevance Pistachio, Template_relevance Bkms_metabolic, Template_relevance Pistachio_ringbreaker, Template_relevance Reaxys, Template_relevance Reaxys_biocatalysis model, leveraging a vast database of chemical reactions to predict feasible synthetic routes.
One-Step Synthesis Focus: Specifically designed for one-step synthesis, it provides concise and direct routes for your target compounds, streamlining the synthesis process.
Accurate Predictions: Utilizing the extensive PISTACHIO, BKMS_METABOLIC, PISTACHIO_RINGBREAKER, REAXYS, REAXYS_BIOCATALYSIS database, our tool offers high-accuracy predictions, reflecting the latest in chemical research and data.
Strategy Settings
Precursor scoring | Relevance Heuristic |
---|---|
Min. plausibility | 0.01 |
Model | Template_relevance |
Template Set | Pistachio/Bkms_metabolic/Pistachio_ringbreaker/Reaxys/Reaxys_biocatalysis |
Top-N result to add to graph | 6 |
Feasible Synthetic Routes
Haftungsausschluss und Informationen zu In-Vitro-Forschungsprodukten
Bitte beachten Sie, dass alle Artikel und Produktinformationen, die auf BenchChem präsentiert werden, ausschließlich zu Informationszwecken bestimmt sind. Die auf BenchChem zum Kauf angebotenen Produkte sind speziell für In-vitro-Studien konzipiert, die außerhalb lebender Organismen durchgeführt werden. In-vitro-Studien, abgeleitet von dem lateinischen Begriff "in Glas", beinhalten Experimente, die in kontrollierten Laborumgebungen unter Verwendung von Zellen oder Geweben durchgeführt werden. Es ist wichtig zu beachten, dass diese Produkte nicht als Arzneimittel oder Medikamente eingestuft sind und keine Zulassung der FDA für die Vorbeugung, Behandlung oder Heilung von medizinischen Zuständen, Beschwerden oder Krankheiten erhalten haben. Wir müssen betonen, dass jede Form der körperlichen Einführung dieser Produkte in Menschen oder Tiere gesetzlich strikt untersagt ist. Es ist unerlässlich, sich an diese Richtlinien zu halten, um die Einhaltung rechtlicher und ethischer Standards in Forschung und Experiment zu gewährleisten.