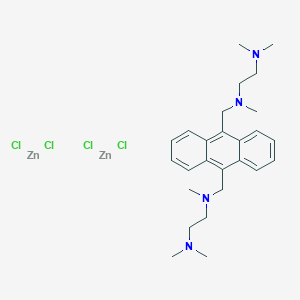
9,10-Bis(TMEDA)anthracene biszinc chloride complex
- Klicken Sie auf QUICK INQUIRY, um ein Angebot von unserem Expertenteam zu erhalten.
- Mit qualitativ hochwertigen Produkten zu einem WETTBEWERBSFÄHIGEN Preis können Sie sich mehr auf Ihre Forschung konzentrieren.
Übersicht
Beschreibung
9,10-Bis(TMEDA)anthracene biszinc chloride complex (9,10-Bis(TMEDA)anthracene-ZnCl2) is an organometallic compound composed of two zinc chloride molecules and a 9,10-bis(TMEDA)anthracene ligand. It is a highly stable complex that is used in a variety of scientific and research applications. This complex has been studied extensively in the fields of organic synthesis, catalysis, and polymer science.
Wissenschaftliche Forschungsanwendungen
Ultrafast Vibrational Dephasing Dynamics
This compound has been used in the study of ultrafast vibrational dephasing dynamics. In a study, 9,10-bis(phenylethynyl)anthracene (BPEA) thin films were revealed by femtosecond coherent anti-stokes Raman scattering spectroscopy (fs-CARS). Dephasing times (T2) of 2.60, 2.98, 3.72, and 1.64 ps were acquired at 1213, 1300, 1400, and 1478 cm−1 vibrations, respectively .
Thermoresponsive Luminescence
The compound has been used in the study of thermoresponsive luminescence. A cyclophane featuring a 9,10-bis(phenylethynyl)anthracene group was designed with shorter flexible oligo(ethyleneglycol) chains used as linkers bridging the luminophore and another aromatic group .
Fluorescence Probe
9,10-Bis(TMEDA)anthracene biszinc chloride complex is widely used as a fluorescence probe in chemical and biological fields .
Photovoltaic Materials
The compound has been used in the development of highly efficient photovoltaic materials .
UV Tracer
Anthracene, a component of this compound, exhibits fluorescence under ultraviolet radiation, making it useful as a UV tracer .
Research Use
The compound is used for research purposes, particularly in the study of its molecular weight and formula .
Eigenschaften
IUPAC Name |
dichlorozinc;N'-[[10-[[2-(dimethylamino)ethyl-methylamino]methyl]anthracen-9-yl]methyl]-N,N,N'-trimethylethane-1,2-diamine |
Source
|
---|---|---|
Source | PubChem | |
URL | https://pubchem.ncbi.nlm.nih.gov | |
Description | Data deposited in or computed by PubChem | |
InChI |
InChI=1S/C26H38N4.4ClH.2Zn/c1-27(2)15-17-29(5)19-25-21-11-7-9-13-23(21)26(20-30(6)18-16-28(3)4)24-14-10-8-12-22(24)25;;;;;;/h7-14H,15-20H2,1-6H3;4*1H;;/q;;;;;2*+2/p-4 |
Source
|
Source | PubChem | |
URL | https://pubchem.ncbi.nlm.nih.gov | |
Description | Data deposited in or computed by PubChem | |
InChI Key |
OGCKUBWDGFVSNZ-UHFFFAOYSA-J |
Source
|
Source | PubChem | |
URL | https://pubchem.ncbi.nlm.nih.gov | |
Description | Data deposited in or computed by PubChem | |
Canonical SMILES |
CN(C)CCN(C)CC1=C2C=CC=CC2=C(C3=CC=CC=C31)CN(C)CCN(C)C.Cl[Zn]Cl.Cl[Zn]Cl |
Source
|
Source | PubChem | |
URL | https://pubchem.ncbi.nlm.nih.gov | |
Description | Data deposited in or computed by PubChem | |
Molecular Formula |
C26H38Cl4N4Zn2 |
Source
|
Source | PubChem | |
URL | https://pubchem.ncbi.nlm.nih.gov | |
Description | Data deposited in or computed by PubChem | |
DSSTOX Substance ID |
DTXSID60550132 |
Source
|
Record name | N~1~,N~1'~-[Anthracene-9,10-diylbis(methylene)]bis(N~1~,N~2~,N~2~-trimethylethane-1,2-diamine)--dichlorozinc (1/2) | |
Source | EPA DSSTox | |
URL | https://comptox.epa.gov/dashboard/DTXSID60550132 | |
Description | DSSTox provides a high quality public chemistry resource for supporting improved predictive toxicology. | |
Molecular Weight |
679.2 g/mol |
Source
|
Source | PubChem | |
URL | https://pubchem.ncbi.nlm.nih.gov | |
Description | Data deposited in or computed by PubChem | |
Product Name |
dichlorozinc;N'-[[10-[[2-(dimethylamino)ethyl-methylamino]methyl]anthracen-9-yl]methyl]-N,N,N'-trimethylethane-1,2-diamine | |
CAS RN |
106682-14-8 |
Source
|
Record name | N~1~,N~1'~-[Anthracene-9,10-diylbis(methylene)]bis(N~1~,N~2~,N~2~-trimethylethane-1,2-diamine)--dichlorozinc (1/2) | |
Source | EPA DSSTox | |
URL | https://comptox.epa.gov/dashboard/DTXSID60550132 | |
Description | DSSTox provides a high quality public chemistry resource for supporting improved predictive toxicology. | |
Record name | N,N'-Bis(2-dimethylaminoethyl)-N,N'-dimethyl-9,10-anthracenedimethanamine biszinc chloride complex | |
Source | European Chemicals Agency (ECHA) | |
URL | https://echa.europa.eu/information-on-chemicals | |
Description | The European Chemicals Agency (ECHA) is an agency of the European Union which is the driving force among regulatory authorities in implementing the EU's groundbreaking chemicals legislation for the benefit of human health and the environment as well as for innovation and competitiveness. | |
Explanation | Use of the information, documents and data from the ECHA website is subject to the terms and conditions of this Legal Notice, and subject to other binding limitations provided for under applicable law, the information, documents and data made available on the ECHA website may be reproduced, distributed and/or used, totally or in part, for non-commercial purposes provided that ECHA is acknowledged as the source: "Source: European Chemicals Agency, http://echa.europa.eu/". Such acknowledgement must be included in each copy of the material. ECHA permits and encourages organisations and individuals to create links to the ECHA website under the following cumulative conditions: Links can only be made to webpages that provide a link to the Legal Notice page. | |
Haftungsausschluss und Informationen zu In-Vitro-Forschungsprodukten
Bitte beachten Sie, dass alle Artikel und Produktinformationen, die auf BenchChem präsentiert werden, ausschließlich zu Informationszwecken bestimmt sind. Die auf BenchChem zum Kauf angebotenen Produkte sind speziell für In-vitro-Studien konzipiert, die außerhalb lebender Organismen durchgeführt werden. In-vitro-Studien, abgeleitet von dem lateinischen Begriff "in Glas", beinhalten Experimente, die in kontrollierten Laborumgebungen unter Verwendung von Zellen oder Geweben durchgeführt werden. Es ist wichtig zu beachten, dass diese Produkte nicht als Arzneimittel oder Medikamente eingestuft sind und keine Zulassung der FDA für die Vorbeugung, Behandlung oder Heilung von medizinischen Zuständen, Beschwerden oder Krankheiten erhalten haben. Wir müssen betonen, dass jede Form der körperlichen Einführung dieser Produkte in Menschen oder Tiere gesetzlich strikt untersagt ist. Es ist unerlässlich, sich an diese Richtlinien zu halten, um die Einhaltung rechtlicher und ethischer Standards in Forschung und Experiment zu gewährleisten.