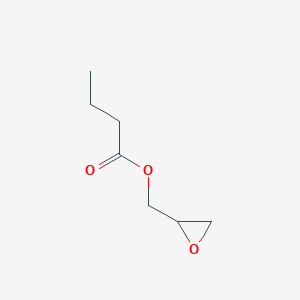
Glycidylbutyrat
Übersicht
Beschreibung
Glycidyl butyrate is an organic compound with the chemical formula C7H12O3. It is a colorless liquid that is soluble in alcohols and ethers but insoluble in water. This compound is widely used as an intermediate in the synthesis of various chemicals, including resins, coatings, and plastics. Its unique chemical structure, which includes an epoxide ring, makes it highly reactive and versatile for various industrial applications .
Wissenschaftliche Forschungsanwendungen
Glycidyl butyrate has a wide range of applications in scientific research, including:
Wirkmechanismus
Target of Action
Glycidyl butyrate, a derivative of butyrate, primarily targets histone deacetylase (HDAC) in the body . HDAC plays a crucial role in the regulation of gene expression through the removal of acetyl groups from histone tails . This process is a key part of epigenetic regulation, which involves changes in gene activity without altering the DNA sequence .
Mode of Action
Glycidyl butyrate interacts with its targets by inhibiting HDAC . This inhibition leads to an increase in histone acetylation, which enhances the accessibility of genes to the transcription machinery . As a result, gene expression is regulated, leading to various physiological effects .
Biochemical Pathways
The primary biochemical pathway affected by glycidyl butyrate is the acetyl-CoA pathway . In this pathway, butyrate, a short-chain fatty acid, is produced through the microbial fermentation of dietary fibers in the colon . Butyrate has been shown to have multiple beneficial effects at both the intestinal and extraintestinal levels . It is also involved in the regulation of lipid and glucose metabolism, inflammation, oxidative stress, and neural signaling .
Pharmacokinetics
Glycidyl butyrate undergoes hydrolysis in the presence of lipase enzyme to form (S)-(-)-glycidol . It may also be used to synthesize 1-butyroyl 2-oleoyl-3-bromo-sn-glycerol and ®-3-(dibenzo[b,e][1,4]dioxin-7-yl)-5-(hydroxymethyl)oxazolidin-2-one . .
Result of Action
The molecular and cellular effects of glycidyl butyrate’s action are primarily related to its role as an HDAC inhibitor . By inhibiting HDAC, glycidyl butyrate can regulate gene expression, leading to various physiological effects . These effects include the regulation of body weight, body composition, and glucose homeostasis . It also has regulatory effects on tissues and cells beyond the colon .
Action Environment
Environmental factors, particularly those related to diet and gut microbiota, can influence the action, efficacy, and stability of glycidyl butyrate . For instance, the production of butyrate is influenced by the composition of the gut microbiota, which in turn is affected by dietary factors . Therefore, a diet rich in fibers that promote the growth of butyrate-producing bacteria can enhance the production and action of butyrate .
Biochemische Analyse
Biochemical Properties
Glycidyl butyrate undergoes hydrolysis in the presence of lipase enzyme to form (S)-(-)-glycidol . This reaction demonstrates the interaction of Glycidyl butyrate with enzymes such as lipase. The nature of this interaction involves the cleavage of the ester bond in Glycidyl butyrate, leading to the formation of (S)-(-)-glycidol .
Cellular Effects
Butyrate has been shown to have significant effects on various types of cells and cellular processes . For instance, it has been found to reduce inflammation in acute gout and lower serum uric acid levels .
Molecular Mechanism
The molecular mechanism of Glycidyl butyrate primarily involves its conversion to (S)-(-)-glycidol through hydrolysis . This reaction is catalyzed by the enzyme lipase . Furthermore, Glycidyl butyrate can be used in the synthesis of polyglycerols through ring-opening polymerization .
Temporal Effects in Laboratory Settings
In laboratory settings, Glycidyl butyrate is used in the controlled synthesis of polyglycerols through ring-opening polymerization . Over time, the pendant butyrate groups in the polymer are readily cleaved by organobase-catalyzed methanolysis, yielding linear polyglycerols .
Dosage Effects in Animal Models
Research on butyrate, a related compound, has shown that it can enhance performance and control gut health disorders in animals when ingested soon after birth .
Metabolic Pathways
It is known that Glycidyl butyrate can be hydrolyzed to form (S)-(-)-glycidol, which suggests its involvement in ester hydrolysis metabolic pathways .
Vorbereitungsmethoden
Synthetic Routes and Reaction Conditions: Glycidyl butyrate can be synthesized through the reaction of butyric acid with glycidol. The reaction typically involves the use of a catalyst, such as sulfuric acid, to facilitate the esterification process. The reaction conditions generally include a temperature range of 60-80°C and a reaction time of several hours to ensure complete conversion .
Industrial Production Methods: In industrial settings, glycidyl butyrate is produced through a similar esterification process but on a larger scale. The reaction is carried out in a continuous flow reactor to optimize production efficiency. The product is then purified through distillation to remove any unreacted starting materials and by-products .
Analyse Chemischer Reaktionen
Types of Reactions: Glycidyl butyrate undergoes several types of chemical reactions, including:
Hydrolysis: In the presence of water and a catalyst, glycidyl butyrate can be hydrolyzed to form butyric acid and glycidol.
Polymerization: It can undergo ring-opening polymerization to form poly(glycidyl butyrate), which is used in the production of various polymers.
Substitution Reactions: The epoxide ring in glycidyl butyrate can react with nucleophiles such as amines, thiols, and acids to form substituted products.
Common Reagents and Conditions:
Hydrolysis: Water and a catalyst such as sulfuric acid or lipase enzyme.
Polymerization: Catalysts such as organobases or metal-free catalysts.
Substitution Reactions: Nucleophiles like amines, thiols, and acids under mild to moderate conditions.
Major Products:
Hydrolysis: Butyric acid and glycidol.
Polymerization: Poly(glycidyl butyrate).
Substitution Reactions: Various substituted glycidyl butyrate derivatives.
Vergleich Mit ähnlichen Verbindungen
Glycidyl methacrylate: Similar in structure but contains a methacrylate group instead of a butyrate group.
Glycidyl acetate: Contains an acetate group instead of a butyrate group.
Uniqueness: Glycidyl butyrate is unique due to its specific reactivity and the properties imparted by the butyrate group. This makes it particularly useful in applications requiring specific chemical and physical properties, such as in the production of flexible and durable polymers .
Eigenschaften
IUPAC Name |
oxiran-2-ylmethyl butanoate | |
---|---|---|
Source | PubChem | |
URL | https://pubchem.ncbi.nlm.nih.gov | |
Description | Data deposited in or computed by PubChem | |
InChI |
InChI=1S/C7H12O3/c1-2-3-7(8)10-5-6-4-9-6/h6H,2-5H2,1H3 | |
Source | PubChem | |
URL | https://pubchem.ncbi.nlm.nih.gov | |
Description | Data deposited in or computed by PubChem | |
InChI Key |
YLNSNVGRSIOCEU-UHFFFAOYSA-N | |
Source | PubChem | |
URL | https://pubchem.ncbi.nlm.nih.gov | |
Description | Data deposited in or computed by PubChem | |
Canonical SMILES |
CCCC(=O)OCC1CO1 | |
Source | PubChem | |
URL | https://pubchem.ncbi.nlm.nih.gov | |
Description | Data deposited in or computed by PubChem | |
Molecular Formula |
C7H12O3 | |
Source | PubChem | |
URL | https://pubchem.ncbi.nlm.nih.gov | |
Description | Data deposited in or computed by PubChem | |
Molecular Weight |
144.17 g/mol | |
Source | PubChem | |
URL | https://pubchem.ncbi.nlm.nih.gov | |
Description | Data deposited in or computed by PubChem | |
CAS No. |
2461-40-7 | |
Record name | Glycidyl butyrate | |
Source | ChemIDplus | |
URL | https://pubchem.ncbi.nlm.nih.gov/substance/?source=chemidplus&sourceid=0002461407 | |
Description | ChemIDplus is a free, web search system that provides access to the structure and nomenclature authority files used for the identification of chemical substances cited in National Library of Medicine (NLM) databases, including the TOXNET system. | |
Record name | 2461-40-7 | |
Source | DTP/NCI | |
URL | https://dtp.cancer.gov/dtpstandard/servlet/dwindex?searchtype=NSC&outputformat=html&searchlist=83144 | |
Description | The NCI Development Therapeutics Program (DTP) provides services and resources to the academic and private-sector research communities worldwide to facilitate the discovery and development of new cancer therapeutic agents. | |
Explanation | Unless otherwise indicated, all text within NCI products is free of copyright and may be reused without our permission. Credit the National Cancer Institute as the source. | |
Synthesis routes and methods I
Procedure details
Synthesis routes and methods II
Procedure details
Synthesis routes and methods III
Procedure details
Synthesis routes and methods IV
Procedure details
Retrosynthesis Analysis
AI-Powered Synthesis Planning: Our tool employs the Template_relevance Pistachio, Template_relevance Bkms_metabolic, Template_relevance Pistachio_ringbreaker, Template_relevance Reaxys, Template_relevance Reaxys_biocatalysis model, leveraging a vast database of chemical reactions to predict feasible synthetic routes.
One-Step Synthesis Focus: Specifically designed for one-step synthesis, it provides concise and direct routes for your target compounds, streamlining the synthesis process.
Accurate Predictions: Utilizing the extensive PISTACHIO, BKMS_METABOLIC, PISTACHIO_RINGBREAKER, REAXYS, REAXYS_BIOCATALYSIS database, our tool offers high-accuracy predictions, reflecting the latest in chemical research and data.
Strategy Settings
Precursor scoring | Relevance Heuristic |
---|---|
Min. plausibility | 0.01 |
Model | Template_relevance |
Template Set | Pistachio/Bkms_metabolic/Pistachio_ringbreaker/Reaxys/Reaxys_biocatalysis |
Top-N result to add to graph | 6 |
Feasible Synthetic Routes
ANone: Glycidyl Butyrate has the molecular formula C₇H₁₂O₃ and a molecular weight of 144.17 g/mol.
A: Gas chromatography [, ] is a widely used technique for the detection and analysis of Glycidyl Butyrate, particularly in reaction mixtures. Researchers often utilize chiral HPLC methods [, ] to determine the enantiomeric purity of Glycidyl Butyrate, especially when evaluating the success of enzymatic resolution processes.
A: Glycidyl Butyrate exists as two enantiomers, (R)-Glycidyl Butyrate and (S)-Glycidyl Butyrate. The (R)-enantiomer is a crucial chiral building block in the synthesis of linezolid [, , ], an oxazolidinone antibiotic. Therefore, efficient methods for separating the enantiomers are crucial for pharmaceutical applications.
A: Enzymatic resolution is a popular approach for separating Glycidyl Butyrate enantiomers. This method utilizes enzymes, specifically lipases [, , , , , , , ], to selectively hydrolyze one enantiomer, leaving the other intact. Porcine pancreatic lipase (PPL) [, , ] has been extensively studied for this purpose.
ANone: Various factors can impact the effectiveness of enzymatic resolution, including:
- Lipase Source: Different lipases exhibit varying degrees of enantioselectivity towards Glycidyl Butyrate [, , ]. Researchers explore various sources, including microorganisms isolated from soil [, , ], to identify lipases with high enantioselectivity.
- Reaction Conditions: Parameters like pH, temperature, substrate concentration, enzyme concentration, and reaction time significantly influence enzyme activity and enantioselectivity [, , , ]. Optimization of these conditions is essential for achieving high enantiomeric purity.
- Reaction Medium: The choice of solvent can impact enzyme activity and enantioselectivity [, ]. Researchers have explored various organic solvents [] and biphasic systems [] to enhance the resolution process.
- Immobilization: Immobilizing enzymes on solid supports can improve their stability and reusability [, ]. Studies have examined the impact of immobilization techniques on the performance of lipases in resolving Glycidyl Butyrate.
A: - Achieving high enantiomeric purity and conversion rates simultaneously can be challenging.- Some studies have reported product inhibition [], where the accumulating product can hinder enzyme activity and affect the resolution process.
A: Yes, researchers have explored chemical synthesis routes for obtaining enantiomerically pure Glycidyl Butyrate. One approach involves the regioselective opening of epichlorohydrin [], a chiral starting material, followed by reactions to introduce the butyrate group. This method can offer advantages in terms of scalability and cost-effectiveness compared to enzymatic resolution.
A: - Polycarbonate Synthesis: Glycidyl Butyrate serves as a monomer in the synthesis of polycarbonates [, ]. These polymers have potential applications as pressure-sensitive adhesives, including in biomedical settings.- Graft Copolymer Synthesis: The epoxide ring in Glycidyl Butyrate makes it amenable to ring-opening reactions with nucleophiles. This reactivity is exploited in the synthesis of graft copolymers [], where Glycidyl Butyrate units within a polymer chain react with functionalized molecules to introduce desired side chains.
Haftungsausschluss und Informationen zu In-Vitro-Forschungsprodukten
Bitte beachten Sie, dass alle Artikel und Produktinformationen, die auf BenchChem präsentiert werden, ausschließlich zu Informationszwecken bestimmt sind. Die auf BenchChem zum Kauf angebotenen Produkte sind speziell für In-vitro-Studien konzipiert, die außerhalb lebender Organismen durchgeführt werden. In-vitro-Studien, abgeleitet von dem lateinischen Begriff "in Glas", beinhalten Experimente, die in kontrollierten Laborumgebungen unter Verwendung von Zellen oder Geweben durchgeführt werden. Es ist wichtig zu beachten, dass diese Produkte nicht als Arzneimittel oder Medikamente eingestuft sind und keine Zulassung der FDA für die Vorbeugung, Behandlung oder Heilung von medizinischen Zuständen, Beschwerden oder Krankheiten erhalten haben. Wir müssen betonen, dass jede Form der körperlichen Einführung dieser Produkte in Menschen oder Tiere gesetzlich strikt untersagt ist. Es ist unerlässlich, sich an diese Richtlinien zu halten, um die Einhaltung rechtlicher und ethischer Standards in Forschung und Experiment zu gewährleisten.