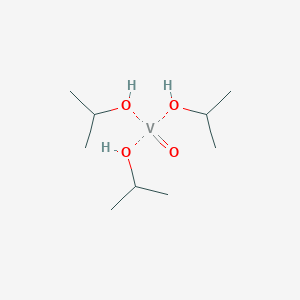
Vanadium(V) oxytriisopropoxide
Übersicht
Beschreibung
Vanadium(V) oxytriisopropoxide (VO(OiPr)₃, CAS 5588-84-1) is an organovanadium compound with the molecular formula C₉H₂₁O₄V and a molecular weight of 244.2 g/mol. It features a vanadium(V) center coordinated by one oxo group (O²⁻) and three isopropoxide ligands (OCH(CH₃)₂), forming a tetrahedral geometry . The compound is a colorless to yellow-green liquid with a density of 1.035 g/mL (25°C) and a boiling point of 80–82°C under reduced pressure (2 mmHg) . It is highly sensitive to moisture and air, requiring storage under inert conditions .
VO(OiPr)₃ is widely used as a precursor in materials science and catalysis due to its solubility in organic solvents (e.g., chloroform, isopropanol) and controlled reactivity. Key applications include:
- Thin-film deposition: Spin-coating for vanadium oxide (V₂O₅) hole-transport layers in organic solar cells .
- Catalysis: Synthesis of supramolecular cage complexes for selective sulfoxidation with turnover numbers (TON) up to 10,000 .
- Hybrid molecular beam epitaxy (hMBE): Growth of vanadium-doped oxide films for electronics .
Vorbereitungsmethoden
Metathesis Reaction: Primary Synthesis Pathway
The most widely employed method for synthesizing VO(OiPr)₃ involves a metathesis reaction between vanadium oxytrichloride (VOCl₃) and sodium isopropoxide (NaOCH(CH₃)₂). This approach leverages the nucleophilic displacement of chloride ligands by isopropoxide groups under anhydrous conditions.
Preparation of Vanadium Oxytrichloride (VOCl₃)
VOCl₃ serves as the foundational precursor for this synthesis. A patented method details its production via the reaction of potassium metavanadate (KVO₃) with concentrated hydrochloric acid (HCl, >30% mass fraction). Key parameters include:
Parameter | Optimal Range | Impact on Yield |
---|---|---|
Molar ratio (KVO₃:HCl) | 1:3.0–5.0 | Maximizes VOCl₃ formation |
Reaction temperature | 50–100°C | Higher temperatures reduce HCl volatility |
Distillation temperature | 70–95°C | Ensures selective separation of VOCl₃ |
Under these conditions, vanadium yields exceed 80%, with distillation achieving purity >99% . The reaction proceeds as:
Stepwise Metathesis with Sodium Isopropoxide
In a rigorously anhydrous environment, VOCl₃ reacts with three equivalents of sodium isopropoxide:
Critical considerations include:
-
Solvent selection : Tetrahydrofuran (THF) or diethyl ether facilitates ligand exchange while minimizing side reactions.
-
Stoichiometry : A 1:3 molar ratio of VOCl₃ to NaOCH(CH₃)₂ ensures complete substitution of chloride ligands .
-
Temperature control : Reactions are conducted at 0–5°C to prevent thermal degradation of the isopropoxide groups.
Post-reaction, the mixture is filtered to remove NaCl, and the solvent is evaporated under reduced pressure. Fractional distillation at 80–82°C yields VO(OiPr)₃ as a colorless to gold liquid .
Alternative Synthesis Routes and Modifications
While the metathesis method dominates industrial and laboratory settings, alternative approaches have been explored for specialized applications.
Direct Alcoholysis of Vanadium Pentoxide
Vanadium pentoxide (V₂O₅) reacts with isopropyl alcohol in the presence of a dehydrating agent (e.g., thionyl chloride):
This method is less favored due to lower yields (~60%) and challenges in removing residual sulfur byproducts.
Reductive Alkoxylation
Vanadium(V) oxide can be reduced with isopropyl alcohol under hydrogen atmosphere, though this method risks over-reduction to lower oxidation states:
Stoichiometric control and catalyst selection (e.g., Pt/C) are critical to maintaining the +5 oxidation state .
Characterization and Quality Control
Ensuring the integrity of VO(OiPr)₃ requires multimodal characterization:
Spectroscopic Analysis
-
FT-IR : Peaks at 985 cm⁻¹ (V=O stretch) and 1120 cm⁻¹ (C-O-V asymmetric stretch) confirm ligand coordination .
-
NMR : ¹H NMR in C₆D₆ shows a septet at δ 4.3 ppm (methine protons) and a doublet at δ 1.2 ppm (methyl groups) .
Elemental and Thermal Analysis
-
Vanadium content : Titration with KMnO₄ yields 20.5–21.3% V, consistent with theoretical values .
-
Thermogravimetric analysis (TGA) : Decomposition initiates at 200°C, correlating with isopropoxide ligand loss .
Industrial-Scale Production Considerations
Scaling VO(OiPr)₃ synthesis introduces challenges distinct from laboratory methods:
Factor | Laboratory Scale | Industrial Scale |
---|---|---|
Atmosphere control | Schlenk lines | Nitrogen-purged reactors |
Purification | Rotary evaporation | Continuous distillation |
Yield optimization | ~70–80% | ~85–90% via recycle streams |
Economic analyses highlight the cost-effectiveness of the metathesis route, with VOCl₃ pricing driving overall expenses .
Emerging Innovations and Research Frontiers
Recent studies focus on tailoring VO(OiPr)₃’s reactivity for catalytic applications:
Analyse Chemischer Reaktionen
Types of Reactions
Triisopropoxyvanadium(V) oxide undergoes various types of chemical reactions, including:
Oxidation: It can act as an oxidizing agent in organic synthesis.
Reduction: It can be reduced to lower oxidation states of vanadium.
Substitution: It can undergo substitution reactions with other ligands[][3].
Common Reagents and Conditions
Common reagents used in reactions with triisopropoxyvanadium(V) oxide include:
Ethanol: Used in substitution reactions.
Acetylacetonate: Used in the formation of neutral oxovanadium complexes.
Major Products Formed
The major products formed from reactions involving triisopropoxyvanadium(V) oxide include:
Vanadium pentoxide: Formed through oxidation reactions.
Neutral oxovanadium complexes: Formed through reactions with ethoxide and acetylacetonate ligands.
Wissenschaftliche Forschungsanwendungen
Catalytic Applications
Vanadium(V) oxytriisopropoxide is primarily recognized for its role as a catalyst in various chemical reactions:
- Asymmetric Transfer Hydrogenation : VTIP serves as a precursor for neutral oxovanadium complexes that are utilized in asymmetric transfer hydrogenation processes, which are crucial for synthesizing chiral compounds .
- Catalysis in Organic Reactions : The compound is employed in several organic transformations, including oxidation reactions and the synthesis of complex organic molecules. Its ability to facilitate reactions under mild conditions makes it valuable in green chemistry .
Energy Storage and Conversion
The potential of VTIP in energy applications is significant:
- Lithium-Ion Batteries : VTIP can be used as a precursor for vanadium oxide materials, which are known to enhance the electrochemical performance of lithium-ion batteries. Research indicates that vanadium-doped materials improve charge capacity and cycling stability .
- Supercapacitors : The compound's unique properties allow it to be integrated into supercapacitor designs, improving energy density and charge/discharge rates due to its high conductivity and structural stability .
Thin Film Deposition
This compound is also utilized in the production of thin films:
- Atomic Layer Deposition (ALD) : VTIP is commonly used as a precursor in ALD processes for creating thin films of vanadium pentoxide (V2O5). These films are important for applications in electronics and optoelectronics, such as sensors and transistors .
- Functional Coatings : The compound can be applied to create protective coatings that enhance the durability and functionality of surfaces in various industrial applications .
Case Study 1: Asymmetric Synthesis Using VTIP
A study demonstrated the effectiveness of vanadium complexes derived from VTIP in asymmetric synthesis, achieving high enantioselectivity in the hydrogenation of ketones. The reaction conditions were optimized to maximize yield while minimizing by-products.
Case Study 2: Vanadium-Doped Lithium-Ion Battery Anodes
Research on lithium-ion batteries incorporating vanadium-doped titanium dioxide showed improved electrochemical performance compared to standard materials. The use of VTIP facilitated the synthesis of the doped material, resulting in higher discharge capacities and better cycling stability over extended use.
Summary Table of Applications
Application Area | Specific Use Case | Benefits |
---|---|---|
Catalysis | Asymmetric transfer hydrogenation | High enantioselectivity |
Energy Storage | Lithium-ion battery anodes | Enhanced charge capacity |
Energy Storage | Supercapacitors | Improved energy density |
Thin Film Deposition | Atomic layer deposition of V2O5 | High-quality thin films |
Functional Coatings | Protective coatings for industrial applications | Increased durability |
Wirkmechanismus
The mechanism of action of triisopropoxyvanadium(V) oxide involves its ability to act as a catalyst in various chemical reactions. It interacts with molecular targets such as organic substrates and promotes the formation of desired products through oxidation, reduction, and substitution reactions. The pathways involved include the formation of intermediate complexes that facilitate the reaction process .
Vergleich Mit ähnlichen Verbindungen
Inorganic Vanadium(V) Precursors
a) Vanadium Pentoxide (V₂O₅)
b) Vanadium Oxytrichloride (VOCl₃)
Key Insight : VOCl₃’s HCl byproduct complicates processing, making VO(OiPr)₃ preferable for environmentally benign applications .
Metal Alkoxide Precursors
a) Titanium(IV) Isopropoxide (TTIP, Ti(OiPr)₄)
Property | VO(OiPr)₃ | TTIP |
---|---|---|
Oxidation State | V⁵⁺ | Ti⁴⁺ |
Decomposition | Forms V₂O₅ via hydrolysis | Forms TiO₂ (anatase/rutile) |
Applications | Vanadium oxide films, catalysis | TiO₂ photovoltaics, photocatalysts |
Key Difference : VO(OiPr)₃’s oxo group stabilizes the +5 oxidation state, enabling unique redox properties absent in TTIP .
b) Zirconium(IV) tert-butoxide (ZTB)
Property | VO(OiPr)₃ | ZTB |
---|---|---|
Thermal Stability | Decomposes at 200°C to VO₄ moieties | Stable up to 300°C (sintering) |
Functionality | Redox-active V⁵⁺/V⁴⁺ transitions | Inert ZrO₂ dielectric films |
Insight : VO(OiPr)₃’s thermal lability is advantageous for low-temperature oxide formation, unlike refractory Zr alkoxides .
Supramolecular Vanadium Complexes
VO(OiPr)₃-derived hemicryptophane cages (e.g., Set II in ) exhibit superior catalytic efficiency (TON = 10,000) in sulfoxidation compared to:
- Open-structure vanadium complexes (Set IV/V): Lower TON due to lack of substrate confinement .
- Small-cavity cages (Set I): Reduced selectivity from steric hindrance .
Mechanistic Advantage : Confinement in VO(OiPr)₃-based cages stabilizes transition states, enabling size-selective catalysis .
Data Table: Comparative Properties of Vanadium Precursors
Biologische Aktivität
Vanadium(V) oxytriisopropoxide (VTIP), an organovanadium compound, has garnered interest in various fields, particularly due to its biological activities and potential applications in medicinal chemistry. This article delves into the biological activity of VTIP, exploring its mechanisms of action, effects on cellular systems, and relevant case studies.
Chemical Structure and Properties
This compound is characterized by the molecular formula and a molecular weight of 244.2 g/mol. In this compound, vanadium is in the +5 oxidation state, coordinated with one oxo group and three isopropoxide ligands. This coordination results in a stable complex that is sensitive to air and moisture, necessitating careful handling and storage under inert conditions .
Oxidative Stress and Lipid Peroxidation
Vanadium compounds, including VTIP, are known to induce oxidative stress by generating reactive oxygen species (ROS). The production of ROS can lead to lipid peroxidation (LPO), a process that damages cellular membranes and alters cellular functions. Studies indicate that vanadium can either exacerbate oxidative damage or exhibit protective effects depending on its concentration and the specific vanadium species involved .
- Lipid Peroxidation Mechanism : LPO involves the oxidation of polyunsaturated fatty acids, leading to the formation of free radicals and secondary products that can further propagate oxidative damage. The balance between ROS production and antioxidant defenses is crucial in determining the overall impact of vanadium compounds on cellular health .
Cytotoxicity and Cell Viability
In vitro studies have demonstrated that VTIP exhibits cytotoxic properties. For instance, research involving organometallic polymers incorporating VTIP showed significant toxicity towards various cell lines. The cytotoxicity was attributed to the leaching of vanadium from the polymer matrix, which resulted in decreased cell viability as measured by MTT assays .
1. Vanadium Compounds and Antioxidant Defense
A notable study highlighted that treatment with certain vanadium complexes could enhance glutathione levels in adipose tissue, suggesting a potential role in antioxidant defense mechanisms. This duality—where vanadium can act both as an oxidative agent and an antioxidant—underscores the complexity of its biological interactions .
2. Cytotoxic Effects in 3D Printed Biomaterials
Research on hybrid organometallic polymer-based biomaterials revealed that while polymers incorporating aluminum and titanium exhibited low toxicity, those containing VTIP were cytotoxic. This finding emphasizes the need for careful evaluation of vanadium-containing materials in biomedical applications .
Summary of Biological Activities
Biological Activity | Effect | Mechanism |
---|---|---|
Oxidative Stress Induction | Increased ROS production | Lipid peroxidation leading to cellular damage |
Cytotoxicity | Decreased cell viability | Leaching of vanadium ions from polymer matrices |
Antioxidant Potential | Enhanced glutathione levels | Modulation of antioxidant defense mechanisms |
Q & A
Basic Research Questions
Q. What are the common synthesis methods for Vanadium(V) oxytriisopropoxide-derived thin films, and how do processing parameters affect film quality?
Methodological Answer: this compound (VTIP) is widely used in vapor-phase deposition techniques like atomic layer deposition (ALD) and chemical vapor deposition (CVD). Key parameters include:
- ALD Cycles : Increasing ALD cycles (200–800 cycles) linearly correlates with film thickness (4–16 nm for V₂O₅) .
- Precursor Reactivity : Oxygen plasma or H₂O₂ enhances ligand exchange during ALD, forming uniform V₂O₅ films .
- Post-Annealing : Annealing ALD-deposited V₂O₅ at 400–500°C in inert/reducing atmospheres converts it to VO₂ or VOx phases .
- Dip-Coating : Solutions of VTIP in isopropanol/acetylacetone yield amorphous V₂O₅ films after annealing at 500°C .
Method | Substrate | Key Parameters | Outcome | Ref. |
---|---|---|---|---|
ALD | SiO₂/Si | 500 cycles, O₂ plasma | 10 nm V₂O₅ | |
CVD | SiO₂/Si | V₂O₅ powder precursor | VO₂ nanobeams | |
Dip-Coating | Porous Si | 3 cycles, 500°C annealing | Crystalline V₂O₅ |
Q. How is this compound utilized in the synthesis of V₂O₅-based materials for energy applications?
Methodological Answer: VTIP is a precursor for V₂O₅ in lithium-ion batteries and solar cells:
- MOF-Templated Synthesis : Reaction with ZIF-67 forms Co₃O₄@Co₃V₂O₈ hollow nanoboxes, enhancing Li-ion storage capacity .
- Hole-Transport Layers (HTLs) : Sol-gel processing of VTIP yields hydrated V₂O₅ layers for organic solar cells, achieving 516 eV XPS peaks (V⁴⁺/V⁵⁺ mix) .
- Hybrid MBE : VTIP serves as an oxygen source for epitaxial LaVO₃ films, enabling stoichiometric growth .
Q. What analytical techniques are critical for characterizing VTIP-grafted surfaces?
Methodological Answer:
- 51V-NMR : Detects grafting efficiency by tracking VTIP-phenol adducts in chloroform .
- DFT Calculations : Models ligand-substrate interactions (e.g., VO₄ sites on SiO₂) .
- Thermogravimetric Analysis (TGA) : Quantifies ligand elimination during pyrolysis (e.g., 2→3 conversion on SiO₂-700) .
Advanced Research Questions
Q. How do ALD parameters influence the catalytic performance of VTIP-derived VOx species in oxidative dehydrogenation (ODH)?
Methodological Answer:
- ALD Cycle Tuning : 200 cycles produce isolated VOx monomers on Al₂O₃, favoring olefin selectivity in cyclohexane ODH. Higher cycles form polyvanadates, promoting benzene formation .
- Substrate Effects : SiO₂-700 supports yield tetrahedral VO₄ sites via SOMC grafting, outperforming traditional impregnated catalysts .
- Thermal Stability : Above 100°C, VTIP decomposes via β-H transfer, necessitating low-temperature ALD for intact VO₄ structures .
Catalyst | ODH Reaction | TON | Selectivity | Ref. |
---|---|---|---|---|
VOx/Al₂O₃ (200 cycles) | Cyclohexane → Olefins | 120 | 85% | |
VO₄/SiO₂-700 | Ethylbenzene → Styrene | 500 | 92% |
Q. What mechanisms explain the size-selective catalytic behavior of VTIP complexes in confined spaces?
Methodological Answer:
- Hemicryptophane Cavities : VTIP-derived cages (Set II) exhibit Michaelis-Menten kinetics in sulfoxidation, with a TON of 10,000 due to transition-state stabilization in 8–10 Å cavities .
- Ligand Design : Binaphthol units in Set II enhance enantioselectivity (up to 90% ee) by restricting substrate orientation .
- Competitive Inhibition : Larger substrates (e.g., thioanisole derivatives) block active sites, reducing activity by 70% .
Q. How can thermal decomposition of VTIP be mitigated during vapor-phase deposition?
Methodological Answer:
- Low-Temperature ALD : Sub-100°C processes with H₂O₂ co-reactants avoid β-H elimination .
- Precursor Pulsing : Short VTIP exposure times (0.1 s pulses) reduce thermal degradation in hybrid MBE .
- Oxygen Gradients : Combinatorial approaches balance VTIP-derived O²⁻ with metallic V fluxes to stabilize VO₂ phases .
Q. Data Contradictions and Resolutions
- Thermal Decomposition Threshold : reports VTIP decomposition >100°C, while uses VTIP at higher temperatures. Resolution: Oxygen-rich environments in combinatorial MBE suppress decomposition .
- V⁴⁺ in V₂O₅ Films : XPS detects V⁴⁺ in hydrated films ( ) but not in crystalline V₂O₅. Resolution: Hydration or organic residues stabilize mixed oxidation states .
Eigenschaften
IUPAC Name |
oxovanadium;propan-2-ol | |
---|---|---|
Source | PubChem | |
URL | https://pubchem.ncbi.nlm.nih.gov | |
Description | Data deposited in or computed by PubChem | |
InChI |
InChI=1S/3C3H8O.O.V/c3*1-3(2)4;;/h3*3-4H,1-2H3;; | |
Source | PubChem | |
URL | https://pubchem.ncbi.nlm.nih.gov | |
Description | Data deposited in or computed by PubChem | |
InChI Key |
JOUSPCDMLWUHSO-UHFFFAOYSA-N | |
Source | PubChem | |
URL | https://pubchem.ncbi.nlm.nih.gov | |
Description | Data deposited in or computed by PubChem | |
Canonical SMILES |
CC(C)O.CC(C)O.CC(C)O.O=[V] | |
Source | PubChem | |
URL | https://pubchem.ncbi.nlm.nih.gov | |
Description | Data deposited in or computed by PubChem | |
Molecular Formula |
C9H24O4V | |
Source | PubChem | |
URL | https://pubchem.ncbi.nlm.nih.gov | |
Description | Data deposited in or computed by PubChem | |
DSSTOX Substance ID |
DTXSID60904393 | |
Record name | Vanadyl triisopropoxide | |
Source | EPA DSSTox | |
URL | https://comptox.epa.gov/dashboard/DTXSID60904393 | |
Description | DSSTox provides a high quality public chemistry resource for supporting improved predictive toxicology. | |
Molecular Weight |
247.23 g/mol | |
Source | PubChem | |
URL | https://pubchem.ncbi.nlm.nih.gov | |
Description | Data deposited in or computed by PubChem | |
Physical Description |
Colorless liquid; [Sigma-Aldrich MSDS] | |
Record name | Vanadium(V) triisopropoxide oxide | |
Source | Haz-Map, Information on Hazardous Chemicals and Occupational Diseases | |
URL | https://haz-map.com/Agents/21834 | |
Description | Haz-Map® is an occupational health database designed for health and safety professionals and for consumers seeking information about the adverse effects of workplace exposures to chemical and biological agents. | |
Explanation | Copyright (c) 2022 Haz-Map(R). All rights reserved. Unless otherwise indicated, all materials from Haz-Map are copyrighted by Haz-Map(R). No part of these materials, either text or image may be used for any purpose other than for personal use. Therefore, reproduction, modification, storage in a retrieval system or retransmission, in any form or by any means, electronic, mechanical or otherwise, for reasons other than personal use, is strictly prohibited without prior written permission. | |
CAS No. |
5588-84-1 | |
Record name | Vanadium, oxotris(2-propanolato)-, (T-4)- | |
Source | ChemIDplus | |
URL | https://pubchem.ncbi.nlm.nih.gov/substance/?source=chemidplus&sourceid=0005588841 | |
Description | ChemIDplus is a free, web search system that provides access to the structure and nomenclature authority files used for the identification of chemical substances cited in National Library of Medicine (NLM) databases, including the TOXNET system. | |
Record name | Vanadium, oxotris(2-propanolato)-, (T-4)- | |
Source | EPA Chemicals under the TSCA | |
URL | https://www.epa.gov/chemicals-under-tsca | |
Description | EPA Chemicals under the Toxic Substances Control Act (TSCA) collection contains information on chemicals and their regulations under TSCA, including non-confidential content from the TSCA Chemical Substance Inventory and Chemical Data Reporting. | |
Record name | Vanadyl triisopropoxide | |
Source | EPA DSSTox | |
URL | https://comptox.epa.gov/dashboard/DTXSID60904393 | |
Description | DSSTox provides a high quality public chemistry resource for supporting improved predictive toxicology. | |
Record name | Oxotris(propan-2-olato)vanadium | |
Source | European Chemicals Agency (ECHA) | |
URL | https://echa.europa.eu/substance-information/-/substanceinfo/100.024.544 | |
Description | The European Chemicals Agency (ECHA) is an agency of the European Union which is the driving force among regulatory authorities in implementing the EU's groundbreaking chemicals legislation for the benefit of human health and the environment as well as for innovation and competitiveness. | |
Explanation | Use of the information, documents and data from the ECHA website is subject to the terms and conditions of this Legal Notice, and subject to other binding limitations provided for under applicable law, the information, documents and data made available on the ECHA website may be reproduced, distributed and/or used, totally or in part, for non-commercial purposes provided that ECHA is acknowledged as the source: "Source: European Chemicals Agency, http://echa.europa.eu/". Such acknowledgement must be included in each copy of the material. ECHA permits and encourages organisations and individuals to create links to the ECHA website under the following cumulative conditions: Links can only be made to webpages that provide a link to the Legal Notice page. | |
Retrosynthesis Analysis
AI-Powered Synthesis Planning: Our tool employs the Template_relevance Pistachio, Template_relevance Bkms_metabolic, Template_relevance Pistachio_ringbreaker, Template_relevance Reaxys, Template_relevance Reaxys_biocatalysis model, leveraging a vast database of chemical reactions to predict feasible synthetic routes.
One-Step Synthesis Focus: Specifically designed for one-step synthesis, it provides concise and direct routes for your target compounds, streamlining the synthesis process.
Accurate Predictions: Utilizing the extensive PISTACHIO, BKMS_METABOLIC, PISTACHIO_RINGBREAKER, REAXYS, REAXYS_BIOCATALYSIS database, our tool offers high-accuracy predictions, reflecting the latest in chemical research and data.
Strategy Settings
Precursor scoring | Relevance Heuristic |
---|---|
Min. plausibility | 0.01 |
Model | Template_relevance |
Template Set | Pistachio/Bkms_metabolic/Pistachio_ringbreaker/Reaxys/Reaxys_biocatalysis |
Top-N result to add to graph | 6 |
Feasible Synthetic Routes
Haftungsausschluss und Informationen zu In-Vitro-Forschungsprodukten
Bitte beachten Sie, dass alle Artikel und Produktinformationen, die auf BenchChem präsentiert werden, ausschließlich zu Informationszwecken bestimmt sind. Die auf BenchChem zum Kauf angebotenen Produkte sind speziell für In-vitro-Studien konzipiert, die außerhalb lebender Organismen durchgeführt werden. In-vitro-Studien, abgeleitet von dem lateinischen Begriff "in Glas", beinhalten Experimente, die in kontrollierten Laborumgebungen unter Verwendung von Zellen oder Geweben durchgeführt werden. Es ist wichtig zu beachten, dass diese Produkte nicht als Arzneimittel oder Medikamente eingestuft sind und keine Zulassung der FDA für die Vorbeugung, Behandlung oder Heilung von medizinischen Zuständen, Beschwerden oder Krankheiten erhalten haben. Wir müssen betonen, dass jede Form der körperlichen Einführung dieser Produkte in Menschen oder Tiere gesetzlich strikt untersagt ist. Es ist unerlässlich, sich an diese Richtlinien zu halten, um die Einhaltung rechtlicher und ethischer Standards in Forschung und Experiment zu gewährleisten.