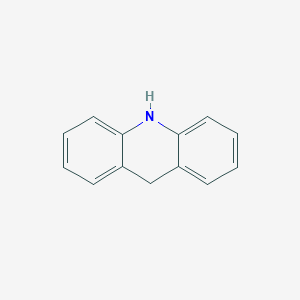
9,10-Dihydroacridine
Übersicht
Beschreibung
9,10-Dihydroacridine, also known as acridan, belongs to the class of organic compounds known as acridines . These are organic compounds containing the acridine moiety, a linear tricyclic heterocycle which consists of two benzene rings joined by a pyridine ring .
Synthesis Analysis
The derivatives of pyridazine and 9,9-dimethyl-9,10-dihydroacridine or phenoxazine were obtained by single-step synthesis employing Buchwald–Hartwig cross-coupling reactions . The compounds are characterized by high thermal stabilities .Molecular Structure Analysis
The 9,10-dihydroacridine molecule contains a total of 30 bond(s). There are 19 non-H bond(s), 13 multiple bond(s), 1 rotatable bond(s), 1 double bond(s), 12 aromatic bond(s), 3 six-membered ring(s), 2 ten-membered ring(s), 1 carboxylic acid(s) (aliphatic), 1 secondary amine(s) (aromatic), and 1 hydroxyl group(s) .Chemical Reactions Analysis
The reactivity of dihydroacridines bearing a С–X fragment at the geminal C-9 atom (where X = C, N, O, P, S) on anode has been investigated by means of electrochemical oxidation and thermodynamic and quantum-chemical calculations . The electrochemical oxidation results either in the formation of the 9-substituted acridines or in the cleavage of the C–X bond .Physical And Chemical Properties Analysis
9,10-Dihydroacridine is a solid under normal conditions . It should be stored under inert gas and away from air as it is air sensitive . Its molecular formula is C13H11N and its molecular weight is 181.24 .Wissenschaftliche Forschungsanwendungen
Organic Light Emitting Diodes (OLEDs)
9,10-Dihydroacridine derivatives are pivotal in the development of OLEDs due to their Thermally Activated Delayed Fluorescence (TADF) properties . These compounds exhibit high thermal stability and are capable of emission from the intramolecular charge transfer state, which is crucial for efficient OLED operation.
Aggregation-Induced Emission (AIE)
The 9,10-Dihydroacridine moiety has been utilized to create AIE-active luminophores . These compounds show enhanced emission in the aggregated state, making them suitable for applications like anti-counterfeiting and information encryption due to their mechanoresponsive luminescence properties.
Photophysical Mechanism Study
The electron-donating properties of 9,10-Dihydroacridine make it an excellent candidate for studying photophysical mechanisms . Its ability to form glass and its positive solvatochromism are of particular interest in understanding the behavior of organic compounds under various conditions.
Anti-Microbial Agents
Derivatives of 9,10-Dihydroacridine have been researched as potential anti-microbial agents . They have shown strong activity against selected Gram-positive bacteria, including MRSA, VISA, and VRE. The compounds disrupt bacterial cell division by interfering with the formation of the Z-ring at the division site, leading to cell death.
Electrochemical Studies
The electrochemical properties of 9,10-Dihydroacridine derivatives are significant for understanding their behavior in electronic applications . Studies involving cyclic voltammetry help in determining their suitability for use in various electronic devices.
Synthesis of Novel Compounds
9,10-Dihydroacridine is a versatile building block for the synthesis of novel compounds with desired photophysical and electrochemical properties . The Buchwald–Hartwig cross-coupling reaction is commonly used to design and synthesize derivatives that can be tailored for specific applications.
Wirkmechanismus
Target of Action
9,10-Dihydroacridine primarily targets DNA and FtsZ, a protein involved in bacterial cell division . The compound’s interaction with DNA is primarily through intercalation, a process where the planar structure of the compound inserts itself between the base pairs of the DNA helix . This interaction with DNA is a common mechanism for many acridine derivatives .
Mode of Action
The mode of action of 9,10-Dihydroacridine involves DNA intercalation and disruption of bacterial cell division . The planar structure of the compound allows it to insert itself between the base pairs of the DNA helix, causing structural changes that can interfere with biological processes involving DNA and related enzymes . In addition, 9,10-Dihydroacridine derivatives have been found to promote FtsZ polymerization and disrupt Z-ring formation at the bacterial cell division site .
Biochemical Pathways
The primary biochemical pathway affected by 9,10-Dihydroacridine is the bacterial cell division process . By promoting FtsZ polymerization and disrupting Z-ring formation, the compound interrupts bacterial cell division, leading to cell death . This mechanism is particularly effective against Gram-positive bacteria, including MRSA, VISA, and VRE .
Result of Action
The result of 9,10-Dihydroacridine’s action is the death of bacterial cells . By disrupting the normal process of bacterial cell division, the compound prevents the bacteria from multiplying, leading to a reduction in the bacterial population .
Safety and Hazards
9,10-Dihydroacridine is classified under GHS07 for safety. It causes skin irritation (H315) and serious eye irritation (H319). Precautionary measures include washing skin thoroughly after handling, wearing protective gloves/eye protection/face protection, and taking off contaminated clothing and washing before reuse .
Zukünftige Richtungen
The derivatives of pyridazine and 9,9-dimethyl-9,10-dihydroacridine or phenoxazine were designed and synthesized by Buchwald–Hartwig cross-coupling reaction . The emission in the range of 534–609 nm of the toluene solutions of the compounds is thermally activated delayed fluorescence with lifetimes of 93 and 143 ns, respectively . This suggests potential applications in the development of efficient organic light-emitting diodes .
Eigenschaften
IUPAC Name |
9,10-dihydroacridine | |
---|---|---|
Source | PubChem | |
URL | https://pubchem.ncbi.nlm.nih.gov | |
Description | Data deposited in or computed by PubChem | |
InChI |
InChI=1S/C13H11N/c1-3-7-12-10(5-1)9-11-6-2-4-8-13(11)14-12/h1-8,14H,9H2 | |
Source | PubChem | |
URL | https://pubchem.ncbi.nlm.nih.gov | |
Description | Data deposited in or computed by PubChem | |
InChI Key |
HJCUTNIGJHJGCF-UHFFFAOYSA-N | |
Source | PubChem | |
URL | https://pubchem.ncbi.nlm.nih.gov | |
Description | Data deposited in or computed by PubChem | |
Canonical SMILES |
C1C2=CC=CC=C2NC3=CC=CC=C31 | |
Source | PubChem | |
URL | https://pubchem.ncbi.nlm.nih.gov | |
Description | Data deposited in or computed by PubChem | |
Molecular Formula |
C13H11N | |
Source | PubChem | |
URL | https://pubchem.ncbi.nlm.nih.gov | |
Description | Data deposited in or computed by PubChem | |
DSSTOX Substance ID |
DTXSID4073887 | |
Record name | Acridine, 9,10-dihydro- | |
Source | EPA DSSTox | |
URL | https://comptox.epa.gov/dashboard/DTXSID4073887 | |
Description | DSSTox provides a high quality public chemistry resource for supporting improved predictive toxicology. | |
Molecular Weight |
181.23 g/mol | |
Source | PubChem | |
URL | https://pubchem.ncbi.nlm.nih.gov | |
Description | Data deposited in or computed by PubChem | |
Product Name |
9,10-Dihydroacridine | |
CAS RN |
92-81-9 | |
Record name | Acridan | |
Source | CAS Common Chemistry | |
URL | https://commonchemistry.cas.org/detail?cas_rn=92-81-9 | |
Description | CAS Common Chemistry is an open community resource for accessing chemical information. Nearly 500,000 chemical substances from CAS REGISTRY cover areas of community interest, including common and frequently regulated chemicals, and those relevant to high school and undergraduate chemistry classes. This chemical information, curated by our expert scientists, is provided in alignment with our mission as a division of the American Chemical Society. | |
Explanation | The data from CAS Common Chemistry is provided under a CC-BY-NC 4.0 license, unless otherwise stated. | |
Record name | Acridan | |
Source | ChemIDplus | |
URL | https://pubchem.ncbi.nlm.nih.gov/substance/?source=chemidplus&sourceid=0000092819 | |
Description | ChemIDplus is a free, web search system that provides access to the structure and nomenclature authority files used for the identification of chemical substances cited in National Library of Medicine (NLM) databases, including the TOXNET system. | |
Record name | Carbazine | |
Source | DTP/NCI | |
URL | https://dtp.cancer.gov/dtpstandard/servlet/dwindex?searchtype=NSC&outputformat=html&searchlist=41239 | |
Description | The NCI Development Therapeutics Program (DTP) provides services and resources to the academic and private-sector research communities worldwide to facilitate the discovery and development of new cancer therapeutic agents. | |
Explanation | Unless otherwise indicated, all text within NCI products is free of copyright and may be reused without our permission. Credit the National Cancer Institute as the source. | |
Record name | Acridine, 9,10-dihydro- | |
Source | EPA DSSTox | |
URL | https://comptox.epa.gov/dashboard/DTXSID4073887 | |
Description | DSSTox provides a high quality public chemistry resource for supporting improved predictive toxicology. | |
Record name | 9,10-dihydroacridine | |
Source | European Chemicals Agency (ECHA) | |
URL | https://echa.europa.eu/substance-information/-/substanceinfo/100.001.994 | |
Description | The European Chemicals Agency (ECHA) is an agency of the European Union which is the driving force among regulatory authorities in implementing the EU's groundbreaking chemicals legislation for the benefit of human health and the environment as well as for innovation and competitiveness. | |
Explanation | Use of the information, documents and data from the ECHA website is subject to the terms and conditions of this Legal Notice, and subject to other binding limitations provided for under applicable law, the information, documents and data made available on the ECHA website may be reproduced, distributed and/or used, totally or in part, for non-commercial purposes provided that ECHA is acknowledged as the source: "Source: European Chemicals Agency, http://echa.europa.eu/". Such acknowledgement must be included in each copy of the material. ECHA permits and encourages organisations and individuals to create links to the ECHA website under the following cumulative conditions: Links can only be made to webpages that provide a link to the Legal Notice page. | |
Record name | ACRIDANE | |
Source | FDA Global Substance Registration System (GSRS) | |
URL | https://gsrs.ncats.nih.gov/ginas/app/beta/substances/ITV7RA4Y9P | |
Description | The FDA Global Substance Registration System (GSRS) enables the efficient and accurate exchange of information on what substances are in regulated products. Instead of relying on names, which vary across regulatory domains, countries, and regions, the GSRS knowledge base makes it possible for substances to be defined by standardized, scientific descriptions. | |
Explanation | Unless otherwise noted, the contents of the FDA website (www.fda.gov), both text and graphics, are not copyrighted. They are in the public domain and may be republished, reprinted and otherwise used freely by anyone without the need to obtain permission from FDA. Credit to the U.S. Food and Drug Administration as the source is appreciated but not required. | |
Synthesis routes and methods I
Procedure details
Synthesis routes and methods II
Procedure details
Retrosynthesis Analysis
AI-Powered Synthesis Planning: Our tool employs the Template_relevance Pistachio, Template_relevance Bkms_metabolic, Template_relevance Pistachio_ringbreaker, Template_relevance Reaxys, Template_relevance Reaxys_biocatalysis model, leveraging a vast database of chemical reactions to predict feasible synthetic routes.
One-Step Synthesis Focus: Specifically designed for one-step synthesis, it provides concise and direct routes for your target compounds, streamlining the synthesis process.
Accurate Predictions: Utilizing the extensive PISTACHIO, BKMS_METABOLIC, PISTACHIO_RINGBREAKER, REAXYS, REAXYS_BIOCATALYSIS database, our tool offers high-accuracy predictions, reflecting the latest in chemical research and data.
Strategy Settings
Precursor scoring | Relevance Heuristic |
---|---|
Min. plausibility | 0.01 |
Model | Template_relevance |
Template Set | Pistachio/Bkms_metabolic/Pistachio_ringbreaker/Reaxys/Reaxys_biocatalysis |
Top-N result to add to graph | 6 |
Feasible Synthetic Routes
Q & A
Q1: What is the molecular formula and weight of 9,10-dihydroacridine?
A1: The molecular formula of 9,10-dihydroacridine is C13H11N, and its molecular weight is 181.23 g/mol. []
Q2: What are some characteristic spectroscopic properties of 9,10-dihydroacridine?
A2: 9,10-Dihydroacridine and its derivatives exhibit strong UV-Vis absorption around 290 nm. [, ] The exact position of absorption maxima can shift depending on the substituents present on the molecule. [] The fluorescence emission of 9,10-dihydroacridines is sensitive to solvent polarity, with pronounced solvatochromic shifts observed for derivatives with electron-donating and electron-withdrawing substituents. []
Q3: How does the crystal structure of 4,9-dimethyl-9,10-dihydroacridine provide insights into its conformation?
A3: Crystallographic analysis of 4,9-dimethyl-9,10-dihydroacridine reveals a non-planar structure, with two aromatic rings connected by sp3 hybridized carbon and nitrogen atoms. [] The angle between the two aromatic planes is not 180°, indicating a bent conformation. This non-planarity plays a crucial role in the molecule's photophysical properties and reactivity.
Q4: What are the thermal stability characteristics of pyridazine-based compounds incorporating 9,9-dimethyl-9,10-dihydroacridine?
A4: Pyridazine derivatives containing 9,9-dimethyl-9,10-dihydroacridine as a donor moiety exhibit high thermal stability, with a 5% weight loss temperature of 314 °C observed for one such compound. [] This high thermal stability is beneficial for applications in organic light-emitting diodes (OLEDs) that require materials to withstand high operational temperatures.
Q5: Can 9,10-dihydroacridine derivatives act as catalysts in organic reactions?
A5: Yes, 9,10-dihydroacridine derivatives, specifically nitroxide radicals, have been investigated as organocatalysts for C-H activation reactions. [] For example, tert-butyl(10-phenyl-9-anthryl)nitroxide effectively catalyzed the oxidative coupling of 9,10-dihydroacridine and nitromethane.
Q6: How do cobalt porphyrins influence the reactivity of 9,10-dihydroacridines with dioxygen?
A6: Monomeric cobalt porphyrins and cofacial dicobalt porphyrins catalyze the two-electron and four-electron reduction of dioxygen by 9,10-dihydroacridines. [] Depending on the 9-substituent on the dihydroacridine, the reaction can proceed via dehydrogenation or oxygenation pathways.
Q7: Can fluorescein act as a photocatalyst for the C(sp3)-H phosphorylation of 9,10-dihydroacridines?
A7: Yes, fluorescein has been successfully employed as a photocatalyst for the C(sp3)-H phosphorylation of 9,10-dihydroacridines using P(O)-H compounds as the phosphorylating agents under blue LED irradiation and an oxygen atmosphere. [] This method provides a convenient approach for synthesizing 9-phosphorylated 9,10-dihydroacridine derivatives.
Q8: Can 9,10-dihydroacridine and its derivatives participate in photochemical reductive acylation reactions?
A8: Yes, under visible light irradiation, 9,10-dihydroacridine, acridine, and 10-methylacridinium salts can undergo reductive acylation in the presence of aldehydes like acetaldehyde or propionaldehyde. [] This reaction results in the formation of N-acylated dihydrophenazines or 9-acyl-9,10-dihydroacridine derivatives.
Q9: How does the nature of the 9-substituent in 9,10-dihydroacridine derivatives influence their electron-transfer oxidation?
A9: The cleavage pathway of the radical cations formed during the electron-transfer oxidation of 9-substituted 10-methyl-9,10-dihydroacridines is highly dependent on the nature of the 9-substituent. [] Electron-donating groups tend to favor C(9)-H bond cleavage, while electron-withdrawing groups promote C(9)-C bond cleavage.
Q10: How do substituents on the acridone ring influence the antitumor activity of acridone-4-acetic acid derivatives?
A10: Studies on acridone-4-acetic acid analogues, structurally related to the antitumor agent xanthenone-4-acetic acid, reveal that substitutions at the 1-, 2-, and 7-positions on the acridone ring are detrimental to activity. [] In contrast, lipophilic substituents at the 5- and 6-positions enhance the ability to induce hemorrhagic necrosis in colon 38 tumors in mice.
Q11: How do chalcogenophosphoryl substituents impact the electrochemical aromatization of 9,10-dihydroacridine derivatives?
A11: Introducing chalcogenophosphoryl groups at the 9-position of 9,10-dihydroacridines significantly influences their anodic oxidation behavior. [] This effect can be attributed to the electronic and steric properties of the chalcogenophosphoryl substituents, as evidenced by X-ray crystallography, computational studies, and cyclic voltammetry measurements.
Q12: Why are 9,10-dihydroacridine derivatives promising materials for OLED applications?
A12: 9,10-dihydroacridine derivatives are explored as host materials in OLEDs due to their high triplet energies, good thermal stability, and efficient charge transport properties. [, , , ] Their ability to efficiently transfer energy to phosphorescent emitters makes them suitable for high-performance red and blue phosphorescent OLEDs.
Q13: How does the linking strategy in 9,10-dihydroacridine-based host materials affect their performance in red phosphorescent OLEDs?
A13: Attaching electron-accepting groups, like benzonitrile or picolinonitrile, to the 4-position of 9,10-dihydroacridine through a phenyl spacer effectively limits conjugation length and maintains high triplet energy levels. [] This approach leads to efficient energy transfer to red phosphorescent emitters, resulting in improved device performance with external quantum efficiencies (EQEs) exceeding 20%.
Q14: What are the advantages of using 9,10-dihydroacridine derivatives with twisted structures as host materials in blue OLEDs?
A14: Twisted 9,10-dihydroacridine derivatives, such as those incorporating dibenzothiophene or dibenzofuran units, possess high triplet energies due to limited conjugation. [] This feature enables efficient energy transfer to blue phosphorescent emitters, leading to high-efficiency blue OLEDs with EQEs exceeding 20%.
Q15: How can the aggregation-induced emission (AIE) property of certain 9,10-dihydroacridine derivatives be leveraged in OLED design?
A15: 9,10-dihydroacridine derivatives exhibiting AIE properties show enhanced luminescence in the solid state, making them suitable for non-doped OLEDs. [] By strategically incorporating exciton-blocking layers, efficient homojunction-like emission from the AIE emitter itself can be achieved, resulting in high-performance sky-blue OLEDs with EQEs reaching 17.2%.
Q16: What is the role of intra- and intermolecular exciplex formation in OLEDs based on meta-linked acridine-triazine conjugates?
A16: Meta-linked acridine-triazine conjugates can form both intra- and intermolecular exciplexes, which act as efficient exciton sources in non-doped TADF OLEDs. [] The extended conjugation and favorable packing in these molecules contribute to efficient intermolecular charge transfer and enhanced device performance with EQEs exceeding 18%.
Q17: How can density functional theory (DFT) calculations be used to understand the properties and reactivity of 9,10-dihydroacridine derivatives?
A17: DFT calculations provide valuable insights into the electronic structure, geometry, and optical properties of 9,10-dihydroacridine derivatives. [, , ] For instance, DFT can be used to predict the energy levels of the highest occupied molecular orbital (HOMO) and lowest unoccupied molecular orbital (LUMO), which are crucial parameters for OLED applications.
Q18: How does computational modeling contribute to the design of 9,10-dihydroacridine-based TADF emitters with improved efficiency?
A18: Theoretical calculations, such as time-dependent DFT (TD-DFT), can be employed to predict the energy gap (ΔEST) between the singlet (S1) and triplet (T1) excited states of TADF emitters. [, ] By systematically modifying the molecular structures and evaluating their calculated ΔEST values, researchers can design 9,10-dihydroacridine-based TADF materials with small ΔEST for efficient reverse intersystem crossing (RISC).
Haftungsausschluss und Informationen zu In-Vitro-Forschungsprodukten
Bitte beachten Sie, dass alle Artikel und Produktinformationen, die auf BenchChem präsentiert werden, ausschließlich zu Informationszwecken bestimmt sind. Die auf BenchChem zum Kauf angebotenen Produkte sind speziell für In-vitro-Studien konzipiert, die außerhalb lebender Organismen durchgeführt werden. In-vitro-Studien, abgeleitet von dem lateinischen Begriff "in Glas", beinhalten Experimente, die in kontrollierten Laborumgebungen unter Verwendung von Zellen oder Geweben durchgeführt werden. Es ist wichtig zu beachten, dass diese Produkte nicht als Arzneimittel oder Medikamente eingestuft sind und keine Zulassung der FDA für die Vorbeugung, Behandlung oder Heilung von medizinischen Zuständen, Beschwerden oder Krankheiten erhalten haben. Wir müssen betonen, dass jede Form der körperlichen Einführung dieser Produkte in Menschen oder Tiere gesetzlich strikt untersagt ist. Es ist unerlässlich, sich an diese Richtlinien zu halten, um die Einhaltung rechtlicher und ethischer Standards in Forschung und Experiment zu gewährleisten.