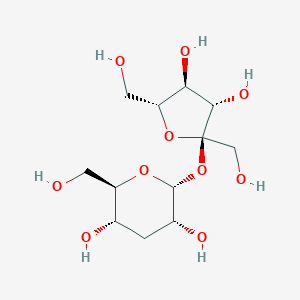
3-Deoxysucrose
Übersicht
Beschreibung
Ethyl 2-piperazin-1-yl-thiazole-4-carboxylate (CAS: 104481-24-5) is a heterocyclic compound with the molecular formula C₁₀H₁₅N₃O₂S and a molecular weight of 241.31 g/mol . It belongs to the thiazole carboxylate family, characterized by a piperazine-substituted thiazole core and an ethyl ester functional group. This compound is commercially available with purities ranging from 95% to >97%, depending on the supplier .
Vorbereitungsmethoden
Synthetic Routes and Reaction Conditions: The synthesis of 3-Deoxysucrose typically involves the selective removal of the hydroxyl group at the third carbon of the glucose unit in sucrose. One common method is the use of glycosyl fluoride donors in the presence of calcium ions and trimethylamine. This reaction proceeds at room temperature in an aqueous solvent mixture, promoting high site-selectivity for the 3’-position of the fructofuranoside unit .
Industrial Production Methods: While specific industrial production methods for this compound are not extensively documented, the principles of glycosylation reactions and the use of selective deoxygenation techniques can be adapted for large-scale synthesis. The use of non-enzymatic aqueous oligosaccharide syntheses is a promising approach for industrial applications .
Analyse Chemischer Reaktionen
Types of Reactions: 3-Deoxysucrose undergoes various chemical reactions, including:
Oxidation: The compound can be oxidized to form corresponding ketones or aldehydes.
Reduction: Reduction reactions can convert this compound into its corresponding alcohols.
Substitution: The hydrogen atom at the third carbon can be substituted with other functional groups, such as halogens or alkyl groups.
Common Reagents and Conditions:
Oxidation: Common oxidizing agents include potassium permanganate (KMnO₄) and chromium trioxide (CrO₃).
Reduction: Sodium borohydride (NaBH₄) and lithium aluminum hydride (LiAlH₄) are frequently used reducing agents.
Substitution: Halogenation reactions often use reagents like thionyl chloride (SOCl₂) or phosphorus tribromide (PBr₃).
Major Products: The major products formed from these reactions depend on the specific reagents and conditions used. For example, oxidation can yield ketones or aldehydes, while reduction can produce alcohols .
Wissenschaftliche Forschungsanwendungen
Introduction to 3-Deoxysucrose
This compound is a modified sugar compound derived from sucrose, characterized by the absence of a hydroxyl group at the 3-position. This alteration significantly impacts its chemical properties and biological applications. The compound has garnered attention for its potential uses in various scientific fields, including microbiology, biochemistry, and agricultural research.
Microbial Research
This compound has been used as a probe in microbial studies to investigate bacterial glycan structures. Its unique properties allow researchers to study bacterial cell envelopes, which are critical for understanding bacterial pathogenesis and developing new antibiotics. For example, studies have demonstrated the utility of deoxy sugars in metabolic profiling of bacterial glycans, aiding in the identification of bacterial species and their pathogenic mechanisms .
Plant Physiology
In plant research, derivatives like 6’-deoxy-6’[18F]fluorosucrose have been synthesized to study sucrose transport mechanisms within plants. These studies utilize radioactive labeling to track the movement of sugars through plant tissues, providing insights into phloem loading and transport dynamics . The application of this compound in this context helps elucidate how plants manage carbohydrate distribution under varying physiological conditions.
Pharmaceutical Research
The unique structural properties of this compound make it a candidate for developing new pharmaceutical agents. Its ability to mimic natural sugars while evading enzymatic degradation allows for the design of drug delivery systems that can target specific cells or tissues without being rapidly metabolized.
Biochemical Studies
Researchers have employed this compound to study enzyme-substrate interactions, particularly focusing on enzymes involved in carbohydrate metabolism. By analyzing how these enzymes interact with deoxy sugars compared to their natural counterparts, scientists can gain insights into enzyme specificity and mechanism .
Case Study 1: Bacterial Glycan Profiling
A study investigated the use of azide-containing analogs of rare deoxy amino sugars, including derivatives of this compound, to probe glycan structures in pathogenic bacteria. The results indicated that these analogs could effectively label bacterial glycans, facilitating a deeper understanding of their roles in virulence .
Case Study 2: Sucrose Transport in Maize
Research utilizing 6’-deoxy-6’[18F]fluorosucrose demonstrated its effectiveness in studying sucrose transport mechanisms in maize plants. The findings revealed that wild-type plants exhibited significantly different transport rates compared to mutants lacking specific sucrose transporter genes, highlighting the importance of these transporters in carbohydrate dynamics .
Wirkmechanismus
The mechanism of action of 3-Deoxysucrose involves its interaction with specific enzymes and molecular targets. The absence of the hydroxyl group at the third carbon alters its binding affinity and reactivity compared to sucrose. This modification can affect glycosylation processes and the formation of glycosidic bonds, influencing various biochemical pathways .
Vergleich Mit ähnlichen Verbindungen
Comparison with Structurally Similar Compounds
Ethyl 2-piperazin-1-yl-thiazole-4-carboxylate shares structural motifs with other piperazine- and thiazole-containing compounds. Below is a comparative analysis of its analogs, focusing on molecular features, purity, and applications.
Thiazole and Piperazine Derivatives
Table 1: Comparison of Selected Piperazine/Thiazole Derivatives
Key Observations:
Thiazole vs. Thiazolidinedione : Unlike pioglitazone hydrochloride (a thiazolidinedione derivative used as an antidiabetic drug), Ethyl 2-piperazin-1-yl-thiazole-4-carboxylate lacks the 2,4-dione ring but retains the thiazole scaffold, which is critical for binding to kinase targets .
Piperazine vs. Piperidine : Piperazine derivatives (e.g., 4524-96-3) exhibit higher solubility compared to piperidine analogs (e.g., 357935-97-8) due to additional nitrogen atoms, making them preferable in drug design for improved bioavailability .
Functional Group Diversity : The ethyl ester group in Ethyl 2-piperazin-1-yl-thiazole-4-carboxylate allows for facile hydrolysis to carboxylic acids, a feature absent in amine-substituted analogs like 4524-96-3 .
Heterocyclic Complexity and Reactivity
Ethyl 2-piperazin-1-yl-thiazole-4-carboxylate is distinguished from more complex heterocycles such as Ethyl 4-(2-(4-(5-(thiophen-3-yl)-1,3,4-oxadiazol-2-yl)piperidin-1-yl)acetyl)piperazine-1-carboxylate (CAS: 1448037-76-0). The latter incorporates an oxadiazole-thiophene system, enhancing π-π stacking interactions but complicating synthesis .
Commercial Availability and Challenges
Ethyl 2-piperazin-1-yl-thiazole-4-carboxylate is listed by suppliers such as Aladdin Scientific, TRC, and Matrix Scientific, with prices ranging from $60/50 mg to $1,235/1 g . However, discontinuation notices from vendors like CymitQuimica highlight supply chain vulnerabilities . In contrast, analogs like 4524-96-3 remain widely available due to simpler synthesis .
Biologische Aktivität
3-Deoxysucrose (3-DS) is a rare sugar that has garnered attention due to its unique biological properties and potential applications in various fields, including medicine and agriculture. This article reviews the biological activity of this compound, focusing on its metabolic pathways, therapeutic potential, and implications for wound healing.
Chemical Structure and Properties
This compound is a disaccharide derived from sucrose by the removal of the hydroxyl group at the C-3 position of the glucose moiety. Its chemical formula is C12H22O11, and it possesses a sweetness profile that is lower than that of sucrose. The absence of the hydroxyl group at C-3 alters its interaction with biological systems, making it a subject of interest in pharmacological studies.
Research indicates that this compound undergoes unique metabolic processes compared to other sugars. It is not readily metabolized by typical glycolytic pathways, which may contribute to its low caloric value and potential as a sugar substitute in diabetic diets. Studies have shown that 3-DS can be incorporated into polysaccharides, influencing their structural properties and biological activities .
Table 1: Comparison of Metabolic Pathways for Sugars
Anticancer Properties
Recent studies have highlighted the potential anticancer properties of this compound. It has been shown to inhibit the proliferation of certain cancer cell lines. For instance, in vitro studies demonstrated that 3-DS can induce apoptosis in cancer cells through mechanisms involving oxidative stress and mitochondrial dysfunction . The sugar moiety appears to enhance the selectivity of anticancer agents by modifying their pharmacokinetic profiles, thereby improving therapeutic efficacy against resistant cancer types.
Wound Healing Applications
One of the most promising applications of this compound is in wound healing. A study investigated the incorporation of deoxy-sugar polymer fibers into a wound healing model. The results indicated that these fibers significantly enhanced the metabolic activity and migratory response of fibroblasts, crucial for effective wound repair . This suggests that 3-DS could be developed into a bioactive wound dressing that promotes tissue regeneration.
Case Studies
- Wound Healing Efficacy : In a controlled study using a two-dimensional scratch assay, fibroblast cells treated with 2-deoxy-D-ribose (2dDR), which shares structural similarities with 3-DS, exhibited increased migration rates compared to controls. This effect was attributed to enhanced metabolic activity facilitated by the sugar's incorporation into polymer matrices .
- Anticancer Activity : A series of experiments conducted on various cancer cell lines revealed that this compound could reduce cell viability significantly at specific concentrations. The mechanism was linked to its ability to interfere with cellular energy metabolism and induce oxidative stress .
Q & A
Basic Research Questions
Q. What are the most reliable methods for synthesizing 3-Deoxysucrose with high purity, and how can potential byproducts be minimized?
- Methodology : Synthesis via glycosylation of unprotected sucrose using glycosyl fluorides in the presence of calcium ions has been reported as effective. Optimization of reaction conditions (e.g., temperature, solvent polarity) and purification via high-performance liquid chromatography (HPLC) can minimize byproducts like 2-deoxysucrose isomers .
- Analytical Validation : Confirm purity using NMR spectroscopy and mass spectrometry (MS) to distinguish between structural isomers .
Q. How can researchers distinguish this compound from its structural isomers (e.g., 2-Deoxysucrose) during characterization?
- Techniques : X-ray crystallography provides definitive structural elucidation. Alternatively, nuclear Overhauser effect spectroscopy (NOESY) NMR can identify spatial proximity of hydroxyl groups unique to this compound .
Q. What standardized protocols exist for assessing the stability of this compound under varying pH and temperature conditions?
- Experimental Design : Use accelerated stability testing (e.g., 40°C/75% relative humidity for 6 months) with periodic sampling. Quantify degradation products via LC-MS and compare against International Council for Harmonisation (ICH) guidelines .
Q. Which in vitro assays are appropriate for preliminary screening of this compound’s biological activity?
- Approach : Enzyme inhibition assays (e.g., glycosidase activity) using spectrophotometric methods. Include positive controls (e.g., deoxy sugars with known activity) and triplicate replicates to ensure reproducibility .
Advanced Research Questions
Q. How can dynamic structural behavior of this compound in aqueous solutions be analyzed to inform its functional interactions?
- Methodology : Employ molecular dynamics (MD) simulations paired with small-angle X-ray scattering (SAXS) to model conformational changes. Validate predictions using -NMR relaxation experiments .
Q. What strategies resolve contradictions in reported bioactivity data for this compound across studies?
- Critical Analysis : Conduct a systematic review with meta-analysis to assess heterogeneity sources (e.g., assay conditions, purity thresholds). Apply statistical tools like funnel plots to detect publication bias .
Q. How can researchers design experiments to investigate synergistic effects between this compound and other bioactive carbohydrates?
- Experimental Framework : Use factorial design (e.g., 2 designs) to test combinatorial effects on cellular uptake or metabolic pathways. Include dose-response matrices and synergy quantification via the Chou-Talalay method .
Q. What advanced spectroscopic techniques are required to address anomalies in this compound’s reported spectral data?
- Resolution : Apply 2D NMR techniques (e.g., HSQC, HMBC) to resolve overlapping signals. Cross-reference with computational NMR prediction tools (e.g., ACD/Labs) .
Q. How can computational models predict this compound’s interaction with membrane transporters or receptors?
- Modeling Workflow : Use molecular docking (AutoDock Vina) and binding free energy calculations (MM/PBSA) to prioritize targets. Validate with surface plasmon resonance (SPR) or isothermal titration calorimetry (ITC) .
Q. Methodological Considerations
Q. How should researchers handle the hygroscopicity of this compound during storage and experimental use?
- Best Practices : Store under inert gas (argon) in desiccators with silica gel. Pre-dry samples via lyophilization before kinetic or thermodynamic studies .
Q. What steps ensure accurate quantification of this compound in complex biological matrices?
- Analytical Rigor : Use isotope dilution mass spectrometry (ID-MS) with -labeled internal standards. Optimize extraction protocols to minimize matrix interference .
Q. How can researchers validate the absence of endotoxins or microbial contaminants in this compound samples used for in vivo studies?
- Quality Control : Perform Limulus amebocyte lysate (LAL) assays for endotoxins and aerobic/anaerobic culture tests. Adhere to Good Laboratory Practice (GLP) guidelines .
Q. Data Presentation and Reproducibility
Q. What are the best practices for visualizing this compound’s structural and functional data in publications?
- Guidelines : Use PyMOL for 3D structural renderings and OriginLab for kinetic plots. Avoid overcrowding figures with excessive chemical structures; prioritize clarity per journal guidelines .
Q. How can researchers enhance reproducibility when reporting synthetic yields of this compound?
- Documentation : Provide detailed reaction logs (e.g., solvent batches, catalyst lot numbers) in supplementary materials. Share raw NMR/MS files in public repositories like Zenodo .
Q. Emerging Research Directions
Q. What mechanistic studies could elucidate this compound’s role in glycocalyx remodeling or cell signaling?
- Proposal : Integrate glycomics and transcriptomics (RNA-seq) in model cell lines. Use CRISPR-Cas9 knockouts of candidate receptors to establish causality .
Q. How can isotopic labeling of this compound advance metabolic flux analysis in living systems?
- Innovation : Synthesize - or -labeled derivatives for tracer studies. Pair with kinetic modeling (e.g., INCA software) to map metabolic pathways .
Eigenschaften
IUPAC Name |
(2R,3R,5S,6R)-2-[(2R,3S,4S,5R)-3,4-dihydroxy-2,5-bis(hydroxymethyl)oxolan-2-yl]oxy-6-(hydroxymethyl)oxane-3,5-diol | |
---|---|---|
Source | PubChem | |
URL | https://pubchem.ncbi.nlm.nih.gov | |
Description | Data deposited in or computed by PubChem | |
InChI |
InChI=1S/C12H22O10/c13-2-7-5(16)1-6(17)11(20-7)22-12(4-15)10(19)9(18)8(3-14)21-12/h5-11,13-19H,1-4H2/t5-,6+,7+,8+,9+,10-,11+,12+/m0/s1 | |
Source | PubChem | |
URL | https://pubchem.ncbi.nlm.nih.gov | |
Description | Data deposited in or computed by PubChem | |
InChI Key |
KKBBKUOGNQMNRX-FCYDWXPZSA-N | |
Source | PubChem | |
URL | https://pubchem.ncbi.nlm.nih.gov | |
Description | Data deposited in or computed by PubChem | |
Canonical SMILES |
C1C(C(OC(C1O)OC2(C(C(C(O2)CO)O)O)CO)CO)O | |
Source | PubChem | |
URL | https://pubchem.ncbi.nlm.nih.gov | |
Description | Data deposited in or computed by PubChem | |
Isomeric SMILES |
C1[C@@H]([C@H](O[C@@H]([C@@H]1O)O[C@@]2([C@H]([C@@H]([C@H](O2)CO)O)O)CO)CO)O | |
Source | PubChem | |
URL | https://pubchem.ncbi.nlm.nih.gov | |
Description | Data deposited in or computed by PubChem | |
Molecular Formula |
C12H22O10 | |
Source | PubChem | |
URL | https://pubchem.ncbi.nlm.nih.gov | |
Description | Data deposited in or computed by PubChem | |
DSSTOX Substance ID |
DTXSID80907008 | |
Record name | Hex-2-ulofuranosyl 3-deoxyhexopyranoside | |
Source | EPA DSSTox | |
URL | https://comptox.epa.gov/dashboard/DTXSID80907008 | |
Description | DSSTox provides a high quality public chemistry resource for supporting improved predictive toxicology. | |
Molecular Weight |
326.30 g/mol | |
Source | PubChem | |
URL | https://pubchem.ncbi.nlm.nih.gov | |
Description | Data deposited in or computed by PubChem | |
CAS No. |
102039-75-8 | |
Record name | 3-Deoxysucrose | |
Source | ChemIDplus | |
URL | https://pubchem.ncbi.nlm.nih.gov/substance/?source=chemidplus&sourceid=0102039758 | |
Description | ChemIDplus is a free, web search system that provides access to the structure and nomenclature authority files used for the identification of chemical substances cited in National Library of Medicine (NLM) databases, including the TOXNET system. | |
Record name | Hex-2-ulofuranosyl 3-deoxyhexopyranoside | |
Source | EPA DSSTox | |
URL | https://comptox.epa.gov/dashboard/DTXSID80907008 | |
Description | DSSTox provides a high quality public chemistry resource for supporting improved predictive toxicology. | |
Retrosynthesis Analysis
AI-Powered Synthesis Planning: Our tool employs the Template_relevance Pistachio, Template_relevance Bkms_metabolic, Template_relevance Pistachio_ringbreaker, Template_relevance Reaxys, Template_relevance Reaxys_biocatalysis model, leveraging a vast database of chemical reactions to predict feasible synthetic routes.
One-Step Synthesis Focus: Specifically designed for one-step synthesis, it provides concise and direct routes for your target compounds, streamlining the synthesis process.
Accurate Predictions: Utilizing the extensive PISTACHIO, BKMS_METABOLIC, PISTACHIO_RINGBREAKER, REAXYS, REAXYS_BIOCATALYSIS database, our tool offers high-accuracy predictions, reflecting the latest in chemical research and data.
Strategy Settings
Precursor scoring | Relevance Heuristic |
---|---|
Min. plausibility | 0.01 |
Model | Template_relevance |
Template Set | Pistachio/Bkms_metabolic/Pistachio_ringbreaker/Reaxys/Reaxys_biocatalysis |
Top-N result to add to graph | 6 |
Feasible Synthetic Routes
Haftungsausschluss und Informationen zu In-Vitro-Forschungsprodukten
Bitte beachten Sie, dass alle Artikel und Produktinformationen, die auf BenchChem präsentiert werden, ausschließlich zu Informationszwecken bestimmt sind. Die auf BenchChem zum Kauf angebotenen Produkte sind speziell für In-vitro-Studien konzipiert, die außerhalb lebender Organismen durchgeführt werden. In-vitro-Studien, abgeleitet von dem lateinischen Begriff "in Glas", beinhalten Experimente, die in kontrollierten Laborumgebungen unter Verwendung von Zellen oder Geweben durchgeführt werden. Es ist wichtig zu beachten, dass diese Produkte nicht als Arzneimittel oder Medikamente eingestuft sind und keine Zulassung der FDA für die Vorbeugung, Behandlung oder Heilung von medizinischen Zuständen, Beschwerden oder Krankheiten erhalten haben. Wir müssen betonen, dass jede Form der körperlichen Einführung dieser Produkte in Menschen oder Tiere gesetzlich strikt untersagt ist. Es ist unerlässlich, sich an diese Richtlinien zu halten, um die Einhaltung rechtlicher und ethischer Standards in Forschung und Experiment zu gewährleisten.