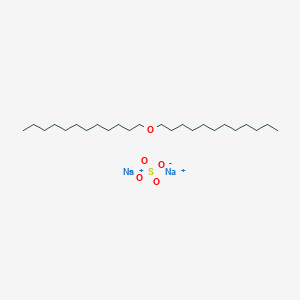
Natriumlaurylsulfat
Übersicht
Beschreibung
It is known for its excellent cleaning and foaming properties, making it a common ingredient in products such as shampoos, soaps, toothpaste, and detergents . The compound consists of a long hydrophobic chain of 12 carbon atoms (lauryl group), a sulfate group, and an ethoxylated chain of variable length .
Wissenschaftliche Forschungsanwendungen
Lauryl ether sulfate sodium has a wide range of scientific research applications:
Vorbereitungsmethoden
Lauryl ether sulfate sodium is synthesized through the ethoxylation of dodecyl alcohol, which is derived from palm kernel oil or coconut oil . The ethoxylation process involves the addition of ethylene oxide to dodecyl alcohol, forming an ethoxylate. This ethoxylate is then converted into a half ester of sulfuric acid and neutralized with sodium hydroxide to produce lauryl ether sulfate sodium . The industrial production process typically involves the following steps:
Ethoxylation: Dodecyl alcohol reacts with ethylene oxide under controlled conditions to form ethoxylated alcohol.
Sulfation: The ethoxylated alcohol is treated with sulfur trioxide or chlorosulfonic acid to form the sulfate ester.
Neutralization: The sulfate ester is neutralized with sodium hydroxide to produce lauryl ether sulfate sodium.
Analyse Chemischer Reaktionen
Lauryl ether sulfate sodium undergoes various chemical reactions, including:
Oxidation: It can be oxidized to form sulfonic acids.
Reduction: Reduction reactions are less common but can occur under specific conditions.
Substitution: It can undergo nucleophilic substitution reactions, where the sulfate group is replaced by other nucleophiles. Common reagents used in these reactions include sulfur trioxide, chlorosulfonic acid, and sodium hydroxide.
Wirkmechanismus
Lauryl ether sulfate sodium acts as a surfactant by lowering the surface tension of water, allowing it to mix more easily with oils and other nonpolar substances . This enables the effective removal of dirt and oil from surfaces. The compound’s amphiphilic nature allows it to align at the interface between water and oil, forming micelles that encapsulate and emulsify nonpolar substances . Additionally, it has protein-denaturing properties and can disrupt cell membranes, making it useful in biological applications .
Vergleich Mit ähnlichen Verbindungen
Lauryl ether sulfate sodium is often compared with other surfactants such as sodium lauryl sulfate and ammonium lauryl sulfate . While all three compounds are effective surfactants, lauryl ether sulfate sodium is less irritating to the skin and eyes compared to sodium lauryl sulfate . This makes it a preferred choice in personal care products. Similar compounds include:
Sodium lauryl sulfate: An anionic surfactant with similar cleaning properties but higher irritation potential.
Ammonium lauryl sulfate: Another anionic surfactant with similar properties but different solubility characteristics.
Sodium pareth sulfate: A surfactant with similar applications but different ethoxylation levels.
Lauryl ether sulfate sodium’s unique combination of effective cleaning, foaming properties, and lower irritation potential makes it a valuable ingredient in various applications.
Biologische Aktivität
Sodium lauryl ether sulfate (SLES) is a widely utilized anionic surfactant found in numerous personal care and cleaning products. Its biological activity is significant due to its effects on microbial communities, potential toxicity, and applications in various fields such as wastewater treatment and pharmaceuticals. This article provides an in-depth examination of the biological activity of SLES, supported by research findings, data tables, and case studies.
SLES is derived from lauryl alcohol through ethoxylation and subsequent sulfation. Its molecular structure allows it to function effectively as a surfactant, lowering the surface tension of water and enhancing solubility of hydrophobic compounds. The general formula for SLES can be represented as:
where represents the average number of ethylene oxide units.
Microbial Degradation of SLES
Research indicates that SLES can be degraded by specific bacterial strains under both aerobic and anaerobic conditions. A study demonstrated that under denitrifying conditions, strains such as Pseudomonas stutzeri and Aeromonas hydrophila effectively degraded concentrations of SLES ranging from 50 mg L to 1000 mg L. Notably, strain S11 was able to degrade 500 mg L SLES in less than one day, showcasing its potential for bioremediation in wastewater treatment processes .
Table 1: Bacterial Strains and Their SLES Degradation Rates
Bacterial Strain | SLES Concentration (mg L) | Degradation Time (days) |
---|---|---|
Pseudomonas stutzeri | 500 | < 1 |
Aeromonas hydrophila | 500 | > 6 |
Pseudomonas nitroreducens | 1000 | < 1 |
Toxicological Studies
Toxicological assessments have been conducted to evaluate the safety profile of SLES. In dermal toxicity studies involving Swiss mice, no significant adverse effects were observed at concentrations up to 5% applied twice weekly . However, irritation studies revealed that concentrations above 5% could induce mild to severe skin irritation in animal models .
Table 2: Skin Irritation Responses to SLES Concentrations
Concentration (%) | Irritation Severity |
---|---|
5 - 5.6 | No irritation |
6 - 26 | Mild erythema |
15 - 30 | Severe irritation |
Applications in Pharmaceuticals
SLES has been investigated for its potential use in pharmaceutical formulations, particularly as a microbicide. A study highlighted its ability to inhibit human sperm motility in vitro, suggesting possible contraceptive applications . Furthermore, formulations containing SLES demonstrated compatibility with latex condoms, indicating its potential utility in reproductive health products.
Case Studies on Environmental Impact
The environmental implications of SLES are significant due to its widespread use and persistence in wastewater systems. A study focusing on the degradation pathways of SLES revealed that while aerobic degradation is well understood, the mechanisms involved in anoxic environments remain less explored. The presence of sulfate ions was identified as a key indicator of successful degradation processes .
Eigenschaften
IUPAC Name |
disodium;1-dodecoxydodecane;sulfate | |
---|---|---|
Source | PubChem | |
URL | https://pubchem.ncbi.nlm.nih.gov | |
Description | Data deposited in or computed by PubChem | |
InChI |
InChI=1S/C24H50O.2Na.H2O4S/c1-3-5-7-9-11-13-15-17-19-21-23-25-24-22-20-18-16-14-12-10-8-6-4-2;;;1-5(2,3)4/h3-24H2,1-2H3;;;(H2,1,2,3,4)/q;2*+1;/p-2 | |
Source | PubChem | |
URL | https://pubchem.ncbi.nlm.nih.gov | |
Description | Data deposited in or computed by PubChem | |
InChI Key |
SMVRDGHCVNAOIN-UHFFFAOYSA-L | |
Source | PubChem | |
URL | https://pubchem.ncbi.nlm.nih.gov | |
Description | Data deposited in or computed by PubChem | |
Canonical SMILES |
CCCCCCCCCCCCOCCCCCCCCCCCC.[O-]S(=O)(=O)[O-].[Na+].[Na+] | |
Source | PubChem | |
URL | https://pubchem.ncbi.nlm.nih.gov | |
Description | Data deposited in or computed by PubChem | |
Molecular Formula |
C24H50Na2O5S | |
Source | PubChem | |
URL | https://pubchem.ncbi.nlm.nih.gov | |
Description | Data deposited in or computed by PubChem | |
Molecular Weight |
496.7 g/mol | |
Source | PubChem | |
URL | https://pubchem.ncbi.nlm.nih.gov | |
Description | Data deposited in or computed by PubChem | |
Physical Description |
Liquid | |
Record name | Poly(oxy-1,2-ethanediyl), .alpha.-sulfo-.omega.-hydroxy-, C10-16-alkyl ethers, sodium salts | |
Source | EPA Chemicals under the TSCA | |
URL | https://www.epa.gov/chemicals-under-tsca | |
Description | EPA Chemicals under the Toxic Substances Control Act (TSCA) collection contains information on chemicals and their regulations under TSCA, including non-confidential content from the TSCA Chemical Substance Inventory and Chemical Data Reporting. | |
CAS No. |
68585-34-2 | |
Record name | Poly(oxy-1,2-ethanediyl), .alpha.-sulfo-.omega.-hydroxy-, C10-16-alkyl ethers, sodium salts | |
Source | EPA Chemicals under the TSCA | |
URL | https://www.epa.gov/chemicals-under-tsca | |
Description | EPA Chemicals under the Toxic Substances Control Act (TSCA) collection contains information on chemicals and their regulations under TSCA, including non-confidential content from the TSCA Chemical Substance Inventory and Chemical Data Reporting. | |
Record name | Alcohols, C10-16, ethoxylated, sulfates, sodium salts | |
Source | European Chemicals Agency (ECHA) | |
URL | https://echa.europa.eu/substance-information/-/substanceinfo/100.105.713 | |
Description | The European Chemicals Agency (ECHA) is an agency of the European Union which is the driving force among regulatory authorities in implementing the EU's groundbreaking chemicals legislation for the benefit of human health and the environment as well as for innovation and competitiveness. | |
Explanation | Use of the information, documents and data from the ECHA website is subject to the terms and conditions of this Legal Notice, and subject to other binding limitations provided for under applicable law, the information, documents and data made available on the ECHA website may be reproduced, distributed and/or used, totally or in part, for non-commercial purposes provided that ECHA is acknowledged as the source: "Source: European Chemicals Agency, http://echa.europa.eu/". Such acknowledgement must be included in each copy of the material. ECHA permits and encourages organisations and individuals to create links to the ECHA website under the following cumulative conditions: Links can only be made to webpages that provide a link to the Legal Notice page. | |
Retrosynthesis Analysis
AI-Powered Synthesis Planning: Our tool employs the Template_relevance Pistachio, Template_relevance Bkms_metabolic, Template_relevance Pistachio_ringbreaker, Template_relevance Reaxys, Template_relevance Reaxys_biocatalysis model, leveraging a vast database of chemical reactions to predict feasible synthetic routes.
One-Step Synthesis Focus: Specifically designed for one-step synthesis, it provides concise and direct routes for your target compounds, streamlining the synthesis process.
Accurate Predictions: Utilizing the extensive PISTACHIO, BKMS_METABOLIC, PISTACHIO_RINGBREAKER, REAXYS, REAXYS_BIOCATALYSIS database, our tool offers high-accuracy predictions, reflecting the latest in chemical research and data.
Strategy Settings
Precursor scoring | Relevance Heuristic |
---|---|
Min. plausibility | 0.01 |
Model | Template_relevance |
Template Set | Pistachio/Bkms_metabolic/Pistachio_ringbreaker/Reaxys/Reaxys_biocatalysis |
Top-N result to add to graph | 6 |
Feasible Synthetic Routes
Haftungsausschluss und Informationen zu In-Vitro-Forschungsprodukten
Bitte beachten Sie, dass alle Artikel und Produktinformationen, die auf BenchChem präsentiert werden, ausschließlich zu Informationszwecken bestimmt sind. Die auf BenchChem zum Kauf angebotenen Produkte sind speziell für In-vitro-Studien konzipiert, die außerhalb lebender Organismen durchgeführt werden. In-vitro-Studien, abgeleitet von dem lateinischen Begriff "in Glas", beinhalten Experimente, die in kontrollierten Laborumgebungen unter Verwendung von Zellen oder Geweben durchgeführt werden. Es ist wichtig zu beachten, dass diese Produkte nicht als Arzneimittel oder Medikamente eingestuft sind und keine Zulassung der FDA für die Vorbeugung, Behandlung oder Heilung von medizinischen Zuständen, Beschwerden oder Krankheiten erhalten haben. Wir müssen betonen, dass jede Form der körperlichen Einführung dieser Produkte in Menschen oder Tiere gesetzlich strikt untersagt ist. Es ist unerlässlich, sich an diese Richtlinien zu halten, um die Einhaltung rechtlicher und ethischer Standards in Forschung und Experiment zu gewährleisten.