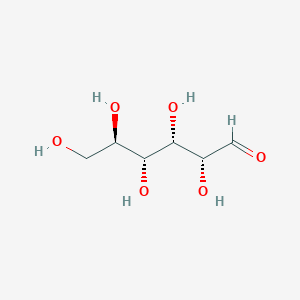
D(+)-Glucose
Übersicht
Beschreibung
D(+)-Glucose, also known as dextrose, is a monosaccharide with the molecular formula C₆H₁₂O₆ and a molecular weight of 180.16 g/mol . It exists as a white crystalline powder, soluble in water, with a melting point of 150–152°C and a density of 1.544 g/cm³ . Its anomeric forms, α-D-glucose and β-D-glucose, differ in the orientation of the hydroxyl group at the C1 position, leading to distinct crystalline structures and physicochemical properties .
This compound is a primary energy source in biological systems, central to glycolysis and cellular respiration. It is used industrially in food additives, pharmaceuticals (e.g., intravenous solutions), and as a metabolic tracer in research .
Vorbereitungsmethoden
Natural Extraction and Hydrolysis of Starch
The most prevalent method for obtaining D(+)-Glucose involves the hydrolysis of starch, a polysaccharide derived from corn, wheat, or potatoes. Starch hydrolysis is typically catalyzed by acids or enzymes (e.g., amylases), breaking α-1,4-glycosidic bonds to yield glucose monomers. Acid hydrolysis employs sulfuric or hydrochloric acid under elevated temperatures (100–150°C), producing a glucose syrup that is subsequently purified via crystallization . Enzymatic hydrolysis, conducted at milder conditions (pH 4.5–6.0, 60–90°C), offers higher specificity and yield, minimizing byproducts like maltose .
Crystallization and Purification
Post-hydrolysis, glucose syrups undergo crystallization to isolate this compound. The process involves supersaturating the solution, seeding with glucose crystals, and cooling to induce crystallization. Ethanol or methanol is often added to reduce solubility, enhancing yield. The crystalline product is then washed with cold solvents to remove impurities, yielding >99% pure this compound .
Synthetic Routes from Glucose Derivatives
While natural extraction dominates industrial production, synthetic methods from glucose derivatives are critical for specialized applications. The provided sources highlight two key intermediates: 3,4,5-tri-O-acetyl D-glucal and 1,2-5,6-diacetone-D-glucose , which are synthesized from this compound or its isomers.
Synthesis of 3,4,5-Tri-O-acetyl D-Glucal
This intermediate, used in the preparation of 2-deoxy-D-glucose , is derived from this compound through acetylation and elimination reactions. This compound is first acetylated using acetic anhydride to protect hydroxyl groups, followed by bromination and β-elimination to form the glucal derivative. Although yields in subsequent hydration steps are low (≈39%), optimizing reaction conditions (e.g., solid acid catalysts) improves efficiency .
Hydration of Glucal Derivatives
3,4,5-Tri-O-acetyl D-glucal undergoes hydration in aqueous media with solid acid catalysts (e.g., sulfonic acid resins) at 20–60°C for 8–24 hours . The reaction proceeds via acid-catalyzed addition of water to the glucal’s double bond, yielding 2-deoxy-D-glucose after deprotection. This method avoids harsh acidic conditions, enhancing environmental sustainability .
Industrial-Scale Production of Diacetone-D-Glucose
The synthesis of 1,2-5,6-diacetone-D-glucose , a chiral intermediate for pharmaceuticals, exemplifies this compound’s role in derivative production . The process involves:
Ketalization with Acetone
α-D-Glucose reacts with acetone in the presence of boron trifluoride etherate (BF₃·Et₂O) at 80–120°C under pressure (2.5–5.5 bar) . The Lewis acid catalyzes the formation of isopropylidene protecting groups, yielding diacetone-D-glucose. Excess acetone is distilled and recycled, ensuring cost-effectiveness.
Purification and Crystallization
Post-reaction, the mixture is neutralized with sodium hydroxide, extracted with dichloromethane, and crystallized from cyclohexane. This yields high-purity diacetone-D-glucose (melting point: 102–104°C), demonstrating the method’s scalability .
Deuterated Glucose Probes for Metabolic Studies
Deuterated D-glucose derivatives, such as 2-deutero-2-deoxy-D-glucose , are synthesized for tracing metabolic pathways . These probes are prepared via selective deuteration of this compound intermediates, enabling studies on glycolysis and hexokinase activity .
Deuteration Techniques
Deuterium is introduced at specific positions (e.g., C-2 or C-6) using deuterated reagents or isotopic exchange. For example, 2-deutero-D-glucose is synthesized by reducing a ketone intermediate with sodium borodeuteride (NaBD₄), achieving >95% isotopic incorporation .
Comparative Analysis of Preparation Methods
Challenges and Innovations in this compound Synthesis
Yield Limitations in Hydration Reactions
The hydration of glucal derivatives often suffers from low yields due to competing side reactions. Recent advances, such as using perfluorinated sulfonic acid resins, have improved yields to 70% while enabling catalyst reuse .
Green Chemistry Approaches
Replacing traditional acids with solid catalysts (e.g., heteropolyacids) reduces waste and energy consumption. For instance, the diacetone-D-glucose process achieves 80% yield with minimal solvent use, aligning with green chemistry principles .
Wissenschaftliche Forschungsanwendungen
Medical Applications
1.1 Metabolic Tracer in Imaging
D(+)-Glucose is increasingly utilized as a non-radioactive tracer in Magnetic Resonance Spectroscopy (MRS). Recent studies have shown that α-D-glucose can be effectively used to measure glucose metabolism in vivo without the risks associated with ionizing radiation. This method allows for longitudinal observational studies of glucose metabolism across the lifespan, making it a promising tool in metabolic research and clinical diagnostics .
1.2 Diabetes Management
This compound plays a crucial role in diabetes management. It is often used in hyperinsulinemic-euglycemic clamp studies to assess insulin sensitivity and glucose metabolism. Research indicates that understanding the pharmacological effects of this compound can lead to improved therapeutic strategies for managing diabetes . Furthermore, it serves as a substrate for developing glycomimetic drugs aimed at ameliorating hyperglycemia and related conditions .
Food Science Applications
2.1 Sweetener and Energy Source
This compound, commonly referred to as dextrose, is widely used as a sweetener in food products. It is favored for its high solubility and sweetness profile, making it suitable for beverages, baked goods, and confectionery items. Its rapid absorption provides a quick source of energy, which is particularly beneficial for athletes during physical exertion .
2.2 Fermentation Processes
In food technology, this compound serves as a key substrate in fermentation processes for producing various food ingredients such as oligosaccharides. For instance, it is utilized in the production of human milk oligosaccharides (HMOs), which are gaining recognition for their health benefits in infant nutrition . The fermentation of this compound leads to the generation of compounds that enhance gut health and immune function.
Biochemical Research Applications
3.1 Glycobiology and Drug Development
This compound is pivotal in glycobiology research, where it aids in understanding glycan structures and their interactions with proteins. This knowledge is essential for drug development, particularly for creating carbohydrate-based therapeutics that target specific diseases .
3.2 Sensing Technologies
Advancements in sensing technologies have leveraged this compound's properties for developing glucose sensors using Near-Infrared Surface-Enhanced Raman Spectroscopy (NIR-SERS). These sensors offer high accuracy and stability for monitoring glucose levels in diabetic patients, facilitating better disease management .
Data Tables
Wirkmechanismus
D-glucose exerts its effects by serving as a primary energy source for cells. It undergoes glycolysis, where it is broken down into pyruvate, generating adenosine triphosphate (ATP) and nicotinamide adenine dinucleotide (NADH) in the process. The generated ATP is used by cells for various energy-requiring processes. D-glucose also participates in the pentose phosphate pathway, which produces ribose-5-phosphate for nucleotide synthesis and NADPH for reductive biosynthesis .
Vergleich Mit ähnlichen Verbindungen
Structural Isomers: α-D-Glucose vs. β-D-Glucose
The α and β anomers of D-glucose exhibit distinct crystalline structures. Experimental and computational studies show differences in atomic coordinates and bond lengths (Table 1). For example, the optimized C1–O1 bond length in α-D-glucose is 1.407 Å, compared to 1.423 Å in β-D-glucose, influencing their stability and reactivity .
Table 1: Structural Parameters of α-D-Glucose and β-D-Glucose
Parameter | α-D-Glucose (Experimental) | β-D-Glucose (Experimental) |
---|---|---|
C1–O1 bond length (Å) | 1.407 | 1.423 |
C2–C3 bond angle (°) | 109.5 | 110.2 |
Crystalline density (g/cm³) | 1.544 | 1.544 |
The β form is more thermodynamically stable in aqueous solutions due to equatorial hydroxyl group orientation, favoring reduced steric hindrance .
Metabolic Analogues: Fructose, Sucrose, and D-Allose
- Fructose : A ketose isomer of glucose, fructose is metabolized independently of insulin, with a lower glycemic index (GI = 19 vs. glucose GI = 100). It is preferentially processed in the liver, contributing to lipogenesis .
- Sucrose: A disaccharide (glucose + fructose) hydrolyzed into monosaccharides by intestinal enzymes. Its GI (65) reflects slower absorption compared to pure glucose .
Table 2: Comparative Metabolic Properties of Hexoses
Compound | Glycemic Index | Primary Metabolic Pathway | Key Functional Difference |
---|---|---|---|
D(+)-Glucose | 100 | Glycolysis, TCA cycle | Insulin-dependent uptake |
Fructose | 19 | Hepatic lipogenesis | Insulin-independent |
D-Allose | N/A | GLUT1 inhibition | Anti-glycolytic activity |
Pharmacological Analogues: Insulin Mimetics and GLUT Inhibitors
- NPC43: A selenium-containing adenosine derivative with a diacetyl ester group at the 2' and 3' positions. It inhibits hepatic glucose production in HepG2 cells (IC₅₀ = 0.8 µM), mimicking insulin without structural similarity to traditional insulin mimetics .
- Compounds #21 and #22: Pyrrolidinone-based inhibitors of GLUT1 (Ki = 1.2 and 0.8 µM, respectively), identified via synthetic lethal screening in glycolytic cancer cells .
Table 3: Inhibitory Effects on Glucose Metabolism
Compound | Target | Mechanism | Efficacy (IC₅₀/Ki) |
---|---|---|---|
NPC43 | Hepatic glucose | Insulin mimetic (selenium-dependent) | 0.8 µM |
Compound #21 | GLUT1 | Competitive inhibition | 1.2 µM |
D-Allose | GLUT1 | Substrate competition | 1.2 µM |
Isotopic Tracers: D-(+)-Glucose-¹³C₆
Stable isotope-labeled glucose (e.g., D-(+)-Glucose-¹³C₆) is used in metabolic flux analysis to trace glycolysis and pentose phosphate pathway activity. Applications include quantifying glucose utilization in cancer cells treated with 2 mM tracer for 8 hours .
Key Research Findings
- Structural Dynamics : β-D-glucose dominates in solution (64% at equilibrium) due to favorable stereoelectronic effects, while α-D-glucose prevails in crystalline states .
- Therapeutic Potential: Rare sugars like D-allose and synthetic inhibitors (e.g., NPC43) offer novel strategies for managing diabetes and cancer .
- Metabolic Reprogramming : GLUT1 inhibitors exploit the Warburg effect in cancer cells, highlighting glucose transport as a therapeutic target .
Biologische Aktivität
D(+)-Glucose, commonly referred to simply as glucose, is a monosaccharide that plays a crucial role in energy metabolism and is fundamental to various biological processes. This article delves into the biological activity of this compound, focusing on its effects on cellular functions, implications in health and disease, and its pharmacological potential.
Overview of this compound
This compound is a simple sugar that serves as a primary energy source for cells. It is involved in several metabolic pathways, including glycolysis, gluconeogenesis, and the pentose phosphate pathway. The compound's structure allows it to participate in various biochemical reactions, influencing cellular metabolism and signaling.
Biological Functions
Energy Metabolism:
- This compound is essential for ATP production through glycolysis, where glucose is converted into pyruvate, yielding energy.
- It also plays a significant role in maintaining blood glucose levels, which are critical for normal physiological functions.
Cell Signaling:
- Glucose acts as a signaling molecule that can influence insulin secretion from pancreatic beta cells.
- It modulates various signaling pathways, including those related to growth factors and stress responses.
Cytotoxic and Genotoxic Effects
Recent studies have highlighted the dual nature of this compound's effects on cells:
-
Cytotoxic Effects:
- High concentrations of this compound (15-25 mM) have been shown to induce cytotoxic effects in cancer cells such as MCF-7 (breast cancer) cells. This effect is characterized by a decrease in cell viability and increased apoptosis rates .
- The MTT assay indicated significant reductions in cell viability at elevated glucose levels, suggesting that hyperglycemia can contribute to tumor cell death .
-
Genotoxic Effects:
- Research has demonstrated that this compound can cause DNA damage in cancer cells. The Comet assay revealed that exposure to high glucose concentrations resulted in increased DNA strand breaks .
- These findings suggest that while glucose is vital for normal cellular function, excessive levels may lead to detrimental effects, particularly in the context of cancer.
Pharmacological Potential
This compound's role extends beyond mere energy provision; it has been explored for its pharmacological properties:
-
Cancer Therapy:
- This compound analogs, such as 2-deoxy-D-glucose (2-DG), have been investigated for their ability to inhibit glycolysis in cancer cells. This inhibition can restrict tumor growth and enhance the efficacy of certain chemotherapeutic agents .
- Studies indicate that 2-DG can selectively target hypoxic tumor cells, making it a promising candidate for cancer treatment .
-
Diabetes Management:
- Understanding the metabolic effects of this compound is crucial for managing diabetes. Elevated glucose levels are associated with oxidative stress and inflammation, contributing to diabetic complications .
- Research into dietary interventions and pharmacological agents aims to modulate glucose metabolism to improve glycemic control in diabetic patients.
Case Studies
Several studies illustrate the biological activity of this compound:
-
Study on Cancer Cell Lines:
A study evaluated the impact of varying concentrations of this compound on MCF-7 cells. Results showed that lower concentrations (5 mM) promoted cell proliferation, while higher concentrations (20-80 mM) led to significant cytotoxicity and apoptosis . -
Metabolic Effects in Diabetic Models:
In animal models of diabetes, oral administration of this compound gel demonstrated alterations in oxidative stress markers and improved metabolic profiles when combined with antioxidants .
Q & A
Basic Research Questions
Q. What experimental methods are recommended for quantifying D(+)-Glucose in cell culture media, and how can cross-reactivity with similar carbohydrates be minimized?
- Methodological Answer : Use enzymatic assays such as the D-Glucose HK Assay Kit (K-GLUHK), which employs hexokinase and glucose-6-phosphate dehydrogenase to specifically convert this compound to NADPH, measurable via spectrophotometry at 340 nm. To avoid cross-reactivity with fructose or galactose, validate the assay using high-performance liquid chromatography (HPLC) with a refractive index detector . Pre-treat samples with glucose oxidase to confirm specificity .
Q. How should researchers handle discrepancies in this compound solubility data across different experimental conditions (e.g., temperature, solvent purity)?
- Methodological Answer : Standardize solvent preparation using anhydrous this compound (≥99.5% purity) and ultrapure water. Document temperature-controlled environments (e.g., 25°C ± 0.5°C) and use Karl Fischer titration to verify water content in solvents. Compare results against the Ph. Eur. or USP monographs for solubility thresholds . Replicate experiments with independent batches to identify systematic errors .
Q. What protocols ensure the stability of this compound in long-term biochemical studies, particularly under varying pH and temperature conditions?
- Methodological Answer : Store this compound in airtight, desiccated containers at -20°C to prevent hygroscopic degradation. For in-solution stability, buffer at pH 6.8–7.2 (phosphate buffer) and avoid prolonged exposure to temperatures >40°C. Monitor degradation products (e.g., 5-hydroxymethylfurfural) via UV-Vis spectroscopy at 284 nm .
Advanced Research Questions
Q. How can isotopic labeling (e.g., ¹³C-D(+)-Glucose) be optimized to trace metabolic flux in cancer cell models without altering native pathways?
- Methodological Answer : Use uniformly labeled ¹³C-D(+)-Glucose at concentrations ≤10 mM to avoid osmotic stress. Validate isotopic incorporation via LC-MS/MS, targeting metabolites like citrate and lactate. Normalize data to unlabeled controls and account for isotopic dilution effects using computational models like Metabolic Flux Analysis (MFA) .
Q. What strategies resolve contradictions in this compound’s role in oxidative stress studies, where it is reported as both pro- and antioxidant?
- Methodological Answer : Context-dependent effects arise from concentration thresholds and cell type variability. For pro-oxidant activity (e.g., glycation), use 25 mM this compound in endothelial cells and measure advanced glycation end-products (AGEs) via ELISA. For antioxidant effects (e.g., NADPH regeneration), employ lower concentrations (5–10 mM) in hepatocytes and quantify glutathione levels .
Q. How do crystallographic polymorphs of this compound (α vs. β anomers) influence its reactivity in enzymatic assays, and how can anomerization be controlled?
- Methodological Answer : Anomerization equilibrium (α:β ≈ 36:64 in solution) can skew kinetic studies. Pre-equilibrate solutions at 37°C for 24 hours or use mutarotase enzyme to accelerate equilibrium. Monitor anomeric ratios via polarimetry ([α]D²⁵ = +52.7° for β-D-Glucose) and validate using ¹H-NMR (δ 5.2 ppm for α-anomer; δ 4.6 ppm for β-anomer) .
Q. What statistical approaches are recommended for analyzing high-throughput this compound uptake data with significant inter-experimental variability?
- Methodological Answer : Apply mixed-effects models to account for batch-to-batch variability. Use principal component analysis (PCA) to identify outliers and hierarchical clustering to group replicates. Normalize data using internal standards (e.g., 2-deoxy-D-glucose) and report uncertainty intervals via bootstrapping .
Q. Data Presentation and Compliance
Q. How should researchers document this compound handling to comply with REACH and EU safety regulations in multidisciplinary studies?
- Methodological Answer : Include CAS No. 50-99-7 and EC No. 200-075-1 in all protocols. For storage, avoid oxidizers and acidic/alkaline materials, and adhere to SEVESO III Directive thresholds. Report safety measures using SDS Section 7 guidelines (e.g., aerosol prevention, waste disposal) .
Q. Tables for Key Methodological Comparisons
Eigenschaften
IUPAC Name |
(3R,4S,5S,6R)-6-(hydroxymethyl)oxane-2,3,4,5-tetrol | |
---|---|---|
Source | PubChem | |
URL | https://pubchem.ncbi.nlm.nih.gov | |
Description | Data deposited in or computed by PubChem | |
InChI |
InChI=1S/C6H12O6/c7-1-2-3(8)4(9)5(10)6(11)12-2/h2-11H,1H2/t2-,3-,4+,5-,6?/m1/s1 | |
Source | PubChem | |
URL | https://pubchem.ncbi.nlm.nih.gov | |
Description | Data deposited in or computed by PubChem | |
InChI Key |
WQZGKKKJIJFFOK-GASJEMHNSA-N | |
Source | PubChem | |
URL | https://pubchem.ncbi.nlm.nih.gov | |
Description | Data deposited in or computed by PubChem | |
Canonical SMILES |
C(C1C(C(C(C(O1)O)O)O)O)O | |
Source | PubChem | |
URL | https://pubchem.ncbi.nlm.nih.gov | |
Description | Data deposited in or computed by PubChem | |
Isomeric SMILES |
C([C@@H]1[C@H]([C@@H]([C@H](C(O1)O)O)O)O)O | |
Source | PubChem | |
URL | https://pubchem.ncbi.nlm.nih.gov | |
Description | Data deposited in or computed by PubChem | |
Molecular Formula |
C6H12O6 | |
Source | PubChem | |
URL | https://pubchem.ncbi.nlm.nih.gov | |
Description | Data deposited in or computed by PubChem | |
DSSTOX Substance ID |
DTXSID501015215, DTXSID901015217 | |
Record name | D-Glucopyranose | |
Source | EPA DSSTox | |
URL | https://comptox.epa.gov/dashboard/DTXSID501015215 | |
Description | DSSTox provides a high quality public chemistry resource for supporting improved predictive toxicology. | |
Record name | Glucopyranose | |
Source | EPA DSSTox | |
URL | https://comptox.epa.gov/dashboard/DTXSID901015217 | |
Description | DSSTox provides a high quality public chemistry resource for supporting improved predictive toxicology. | |
Molecular Weight |
180.16 g/mol | |
Source | PubChem | |
URL | https://pubchem.ncbi.nlm.nih.gov | |
Description | Data deposited in or computed by PubChem | |
CAS No. |
2280-44-6, 54-17-1, 26655-34-5, 1227096-09-4 | |
Record name | D-Glucopyranose | |
Source | CAS Common Chemistry | |
URL | https://commonchemistry.cas.org/detail?cas_rn=2280-44-6 | |
Description | CAS Common Chemistry is an open community resource for accessing chemical information. Nearly 500,000 chemical substances from CAS REGISTRY cover areas of community interest, including common and frequently regulated chemicals, and those relevant to high school and undergraduate chemistry classes. This chemical information, curated by our expert scientists, is provided in alignment with our mission as a division of the American Chemical Society. | |
Explanation | The data from CAS Common Chemistry is provided under a CC-BY-NC 4.0 license, unless otherwise stated. | |
Record name | Glucopyranose | |
Source | CAS Common Chemistry | |
URL | https://commonchemistry.cas.org/detail?cas_rn=54-17-1 | |
Description | CAS Common Chemistry is an open community resource for accessing chemical information. Nearly 500,000 chemical substances from CAS REGISTRY cover areas of community interest, including common and frequently regulated chemicals, and those relevant to high school and undergraduate chemistry classes. This chemical information, curated by our expert scientists, is provided in alignment with our mission as a division of the American Chemical Society. | |
Explanation | The data from CAS Common Chemistry is provided under a CC-BY-NC 4.0 license, unless otherwise stated. | |
Record name | D-Glucopyranose | |
Source | ChemIDplus | |
URL | https://pubchem.ncbi.nlm.nih.gov/substance/?source=chemidplus&sourceid=0002280446 | |
Description | ChemIDplus is a free, web search system that provides access to the structure and nomenclature authority files used for the identification of chemical substances cited in National Library of Medicine (NLM) databases, including the TOXNET system. | |
Record name | D-Glucopyranose | |
Source | EPA DSSTox | |
URL | https://comptox.epa.gov/dashboard/DTXSID501015215 | |
Description | DSSTox provides a high quality public chemistry resource for supporting improved predictive toxicology. | |
Record name | Glucopyranose | |
Source | EPA DSSTox | |
URL | https://comptox.epa.gov/dashboard/DTXSID901015217 | |
Description | DSSTox provides a high quality public chemistry resource for supporting improved predictive toxicology. | |
Record name | D-glucose | |
Source | European Chemicals Agency (ECHA) | |
URL | https://echa.europa.eu/substance-information/-/substanceinfo/100.017.195 | |
Description | The European Chemicals Agency (ECHA) is an agency of the European Union which is the driving force among regulatory authorities in implementing the EU's groundbreaking chemicals legislation for the benefit of human health and the environment as well as for innovation and competitiveness. | |
Explanation | Use of the information, documents and data from the ECHA website is subject to the terms and conditions of this Legal Notice, and subject to other binding limitations provided for under applicable law, the information, documents and data made available on the ECHA website may be reproduced, distributed and/or used, totally or in part, for non-commercial purposes provided that ECHA is acknowledged as the source: "Source: European Chemicals Agency, http://echa.europa.eu/". Such acknowledgement must be included in each copy of the material. ECHA permits and encourages organisations and individuals to create links to the ECHA website under the following cumulative conditions: Links can only be made to webpages that provide a link to the Legal Notice page. | |
Record name | α-D-Glucopyranose | |
Source | European Chemicals Agency (ECHA) | |
URL | https://echa.europa.eu/information-on-chemicals | |
Description | The European Chemicals Agency (ECHA) is an agency of the European Union which is the driving force among regulatory authorities in implementing the EU's groundbreaking chemicals legislation for the benefit of human health and the environment as well as for innovation and competitiveness. | |
Explanation | Use of the information, documents and data from the ECHA website is subject to the terms and conditions of this Legal Notice, and subject to other binding limitations provided for under applicable law, the information, documents and data made available on the ECHA website may be reproduced, distributed and/or used, totally or in part, for non-commercial purposes provided that ECHA is acknowledged as the source: "Source: European Chemicals Agency, http://echa.europa.eu/". Such acknowledgement must be included in each copy of the material. ECHA permits and encourages organisations and individuals to create links to the ECHA website under the following cumulative conditions: Links can only be made to webpages that provide a link to the Legal Notice page. | |
Record name | D-Glucose | |
Source | European Chemicals Agency (ECHA) | |
URL | https://echa.europa.eu/information-on-chemicals | |
Description | The European Chemicals Agency (ECHA) is an agency of the European Union which is the driving force among regulatory authorities in implementing the EU's groundbreaking chemicals legislation for the benefit of human health and the environment as well as for innovation and competitiveness. | |
Explanation | Use of the information, documents and data from the ECHA website is subject to the terms and conditions of this Legal Notice, and subject to other binding limitations provided for under applicable law, the information, documents and data made available on the ECHA website may be reproduced, distributed and/or used, totally or in part, for non-commercial purposes provided that ECHA is acknowledged as the source: "Source: European Chemicals Agency, http://echa.europa.eu/". Such acknowledgement must be included in each copy of the material. ECHA permits and encourages organisations and individuals to create links to the ECHA website under the following cumulative conditions: Links can only be made to webpages that provide a link to the Legal Notice page. | |
Retrosynthesis Analysis
AI-Powered Synthesis Planning: Our tool employs the Template_relevance Pistachio, Template_relevance Bkms_metabolic, Template_relevance Pistachio_ringbreaker, Template_relevance Reaxys, Template_relevance Reaxys_biocatalysis model, leveraging a vast database of chemical reactions to predict feasible synthetic routes.
One-Step Synthesis Focus: Specifically designed for one-step synthesis, it provides concise and direct routes for your target compounds, streamlining the synthesis process.
Accurate Predictions: Utilizing the extensive PISTACHIO, BKMS_METABOLIC, PISTACHIO_RINGBREAKER, REAXYS, REAXYS_BIOCATALYSIS database, our tool offers high-accuracy predictions, reflecting the latest in chemical research and data.
Strategy Settings
Precursor scoring | Relevance Heuristic |
---|---|
Min. plausibility | 0.01 |
Model | Template_relevance |
Template Set | Pistachio/Bkms_metabolic/Pistachio_ringbreaker/Reaxys/Reaxys_biocatalysis |
Top-N result to add to graph | 6 |
Feasible Synthetic Routes
Haftungsausschluss und Informationen zu In-Vitro-Forschungsprodukten
Bitte beachten Sie, dass alle Artikel und Produktinformationen, die auf BenchChem präsentiert werden, ausschließlich zu Informationszwecken bestimmt sind. Die auf BenchChem zum Kauf angebotenen Produkte sind speziell für In-vitro-Studien konzipiert, die außerhalb lebender Organismen durchgeführt werden. In-vitro-Studien, abgeleitet von dem lateinischen Begriff "in Glas", beinhalten Experimente, die in kontrollierten Laborumgebungen unter Verwendung von Zellen oder Geweben durchgeführt werden. Es ist wichtig zu beachten, dass diese Produkte nicht als Arzneimittel oder Medikamente eingestuft sind und keine Zulassung der FDA für die Vorbeugung, Behandlung oder Heilung von medizinischen Zuständen, Beschwerden oder Krankheiten erhalten haben. Wir müssen betonen, dass jede Form der körperlichen Einführung dieser Produkte in Menschen oder Tiere gesetzlich strikt untersagt ist. Es ist unerlässlich, sich an diese Richtlinien zu halten, um die Einhaltung rechtlicher und ethischer Standards in Forschung und Experiment zu gewährleisten.