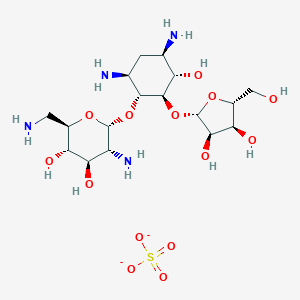
Ribostamycin sulfate
Übersicht
Beschreibung
Ribostamycin sulfate is the sulfate salt form of ribostamycin, an aminoglycoside antibiotic produced by Streptomyces ribosidificus . Its chemical formula is C₁₇H₃₄N₄O₁₀·xH₂SO₄ (molecular weight: ~552.55 g/mol), and it exists as a white to yellowish powder with high solubility in water (100 mg/mL at 25°C) . The sulfate salt enhances solubility and bioavailability, making it suitable for intramuscular or intravenous administration .
Mechanism of Action: this compound binds to the 30S ribosomal subunit, disrupting mRNA translation initiation and causing misreading of genetic codons. This inhibits bacterial protein synthesis, leading to bactericidal effects . It exhibits broad-spectrum activity against Gram-negative bacteria (e.g., Escherichia coli, Klebsiella spp.) and some Gram-positive pathogens . Notably, it retains efficacy against gentamicin-resistant strains by evading aminoglycoside-modifying enzymes .
Vorbereitungsmethoden
Biosynthetic Production via Microbial Fermentation
Ribostamycin is naturally produced by Streptomyces ribosifidicus through a biosynthetic pathway involving glycosylation and amination steps . While industrial-scale fermentation remains a primary production method, details of the microbial pathway are less documented in peer-reviewed literature compared to synthetic approaches. The process typically involves:
-
Cultivation of Streptomyces strains in optimized media to promote antibiotic synthesis.
-
Extraction and purification via ion-exchange chromatography (e.g., Amberlite CG50) and sulfate salt precipitation .
However, challenges in yield consistency and purification efficiency have driven interest in chemical synthesis.
Chemical Synthesis Approaches
Dearomative Hydroamination of Benzene
A landmark 2022 study demonstrated a 10-step synthesis of ribostamycin from benzene, achieving gram-scale production . Key steps include:
-
Catalytic enantioselective hydroamination : Benzene undergoes nickel-catalyzed hydroamination with a urazole-based arenophile to yield cyclohexadienylamine 13 (45% yield, 92% ee) .
-
Temperature control : Scaling to 100 mmol resulted in enantioselectivity erosion (−50°C optimal), highlighting the sensitivity of chiral induction .
Table 1: Key Reactions in Ribostamycin Synthesis
Step | Reaction | Reagents/Conditions | Yield | Selectivity |
---|---|---|---|---|
1 | Hydroamination | Ni(cod)₂, (R)-BINAP, −78°C | 45% | 92% ee |
2 | Epoxidation | m-CPBA, CH₂Cl₂, −20°C | 78% | anti-epoxide |
3 | Glycosylation | Lawesson’s reagent, AgClO₄ | 62% | β-selectivity |
Epoxidation and Cyclization
Following hydroamination, Boc protection and epoxidation of diene 13 yield allylic epoxide 28 as a single diastereomer . The syn-1,3-urazole tether enforces conformational rigidity, enabling regioselective 5-exo-tet cyclization to form the 2-deoxystreptamine (2-DOS) core .
Glycosylation and Deprotection Strategies
-
β-Glycosylation : Ribose donor 32 couples to the 2-DOS core using a thiophilic activator (Lawesson’s reagent/AgClO₄), achieving exclusive β-selectivity .
-
Global deprotection : Hydrogenation over Pearlman’s catalyst (Pd(OH)₂/C) removes six benzyl groups and reduces azides to amines, yielding this compound after ion exchange .
Enzymatic Modification Techniques
While enzymatic synthesis of ribostamycin itself is unreported, related aminoglycosides like neomycin C are prepared from ribostamycin via:
-
Glycosyltransferase (NeoF) : Transfers GlcNAc from UDP-GlcNAc to ribostamycin’s 6′′′-OH .
-
Deacetylase (NeoD) : Hydrolyzes the GlcNAc acetyl group to yield 6′′′-deamino-6′′′-hydroxyneomycin C .
These methods highlight ribostamycin’s utility as a precursor for complex aminoglycosides.
Analytical Characterization and Quality Control
Structural validation of synthetic this compound employs:
-
HR-FAB-MS : m/z 637.3033 ([M+Na]⁺) confirms molecular formula C₂₃H₄₆N₆O₁₃Na .
-
NMR spectroscopy : ¹H and ¹³C NMR (Figure 2 in ) verify stereochemistry and glycosidic linkages.
-
HPLC with UV detection : Dinitrophenyl derivatization ensures purity >95% .
Comparative Evaluation of Synthesis Pathways
Table 2: Biosynthetic vs. Chemical Synthesis
Industrial-Scale Production Considerations
Analyse Chemischer Reaktionen
Resistance Enzymes ( ):
- APH(2'')-Ia kinase : Phosphorylates ribostamycin at the 2''-OH group, reducing ribosomal binding affinity. Structural studies reveal two binding conformations:
- Ribose-down: Minimal enzyme conformational change (PDB: 6BGV).
- Ribose-up: Helical subdomain closure, similar to neomycin binding (PDB: 6BGX).
Derivatives ( ):
- 6'-N-Acetyl-ribostamycin : Reduces ribosomal binding (IC₅₀ = 0.75 μM vs. 0.15 μM for parent).
- 3-N-Acetyl-ribostamycin : Minimal impact on subunit rotation dynamics (IC₅₀ = 34 μM).
Ribosomal Binding and Mechanism of Action
Ribostamycin sulfate interacts with bacterial ribosomes via:
Binding Sites ( ):
Subunit | Interaction | Impact |
---|---|---|
30S (h44) | Hydrogen bonds with A1408, G1491 | Misreading of mRNA |
50S (H69) | Electrostatic interactions with phosphate backbone | Inhibition of translocation |
Key Structural Motifs :
- DOS core : Critical for h44 major groove recognition.
- Ribose moiety : Stabilizes H69 interaction at neutral pH.
Antiviral and Off-Target Interactions
Recent studies highlight non-antibiotic applications:
Protein Disulfide Isomerase (PDI) Inhibition ( ):
- Binding : Ribostamycin competes with LGALS9 at PDI’s thioredoxin-like domain (Kd = 1.2 μM).
- Impact : Alters disulfide bond dynamics in viral glycoproteins (e.g., CHIKV E1/E2) .
Hydrolytic Degradation ( ):
Condition | Degradation Pathway | Half-Life |
---|---|---|
pH < 3 | Cleavage of glycosidic bonds | 2.1 h |
pH 7.4 | Minimal degradation | >48 h |
pH > 10 | Oxidation of amine groups | 6.8 h |
Spectroscopic Data ( ):
Parameter | Value |
---|---|
HR-FAB-MS | m/z 637.3033 [M+Na]⁺ |
¹H-NMR (D₂O) | δ 5.32 (H1', J = 3.5 Hz), δ 3.89 (H6, br s) |
Specific Rotation | [α]²⁰/D +44° (c = 1, H₂O) |
Wissenschaftliche Forschungsanwendungen
Antibacterial Activity
Ribostamycin exhibits significant antibacterial properties against both Gram-positive and Gram-negative bacteria. Its mechanism of action primarily involves binding to the bacterial 30S ribosomal subunit, which inhibits protein synthesis by causing misreading of mRNA and blocking the translocation of peptidyl-tRNA .
Minimum Inhibitory Concentration (MIC) Studies
Recent studies have evaluated the MIC of ribostamycin against various pathogens:
The combination of ribostamycin with ethylenediaminetetraacetic acid (EDTA) has shown an 8-fold enhancement in potency against certain strains of E. coli, indicating a promising strategy for overcoming antibiotic resistance .
Clinical Applications
This compound is primarily utilized in treating a variety of infections, particularly in hospital settings. Its applications include:
- Sepsis
- Skin Infections : Superficial and deep skin infections, including chronic pyoderma and osteomyelitis.
- Respiratory Infections : Acute bronchitis, pneumonia, pulmonary abscess.
- Urinary Tract Infections : Cystitis and pyelonephritis.
- Other Infections : Gonococcal infections, peritonitis, cholecystitis, dacryocystitis, keratitis, otitis media, sinusitis .
Pharmacological Insights
Ribostamycin is classified as a critically important antimicrobial by the World Health Organization due to its effectiveness against resistant bacterial strains. Its pharmacological profile includes:
Property | Details |
---|---|
Target Mechanism | Binds to 30S ribosomal subunit |
Broad-Spectrum Activity | Effective against various bacterial pathogens |
Adverse Reactions | Renal dysfunction, liver disorder, rash |
Research Developments
Recent research has focused on enhancing the clinical efficacy of ribostamycin through combination therapies and novel delivery methods. Studies indicate that ribostamycin can be effectively combined with other agents like EDTA to improve its antibacterial spectrum and reduce resistance .
Case Studies
Several case studies have documented the successful use of ribostamycin in treating resistant infections:
- A study reported the use of ribostamycin in a patient with chronic respiratory lesions who showed significant improvement after treatment.
- Another case highlighted its role in managing complicated skin infections where conventional antibiotics failed.
These cases underscore the potential of ribostamycin as a viable option for treating difficult-to-manage infections.
Wirkmechanismus
Ribostamycin-Sulfat entfaltet seine Wirkung, indem es an die bakterielle 30S-Ribosomenuntereinheit bindet und die Translokation des Peptidyl-tRNA vom A- zum P-Standort hemmt . Diese Bindung führt zu einem Fehllesen der mRNA, wodurch das Bakterium nicht in der Lage ist, für sein Wachstum wichtige Proteine zu synthetisieren . Der genaue Wirkungsmechanismus umfasst mehrere molekulare Ziele, darunter Proteindisulfid-Isomerase und verschiedene ribosomale Proteine .
Vergleich Mit ähnlichen Verbindungen
Unique Features :
- Lower Nephrotoxicity and Ototoxicity: Compared to other aminoglycosides, ribostamycin sulfate demonstrates reduced renal and auditory toxicity in animal models .
- PDI Molecular Chaperone Inhibition: At a molar ratio of 100:1, this compound inhibits protein disulfide isomerase (PDI) chaperone activity, a mechanism distinct from classical aminoglycosides .
Structural and Pharmacological Differences
Table 1: Key Properties of this compound and Comparable Aminoglycosides
Compound | Molecular Weight (g/mol) | Solubility (Water) | Key Targets | MIC Range (mg/L)* |
---|---|---|---|---|
This compound | 552.55 | 100 mg/mL | 30S ribosomal subunit, PDI | 18.7 (vs Borrelia spp.) |
Gentamicin | 477.60 | 50 mg/mL | 30S ribosomal subunit | 0.5–4 (vs Pseudomonas) |
Kanamycin | 484.50 | 50 mg/mL | 30S ribosomal subunit | 1–8 (vs Mycobacteria) |
Amikacin | 585.60 | 50 mg/mL | 30S ribosomal subunit | 1–16 (vs MDR Gram-negative) |
Tobramycin | 467.52 | 50 mg/mL | 30S ribosomal subunit | 0.25–2 (vs Acinetobacter) |
*MIC = Minimum Inhibitory Concentration. Data derived from .
Toxicity Profile
Table 2: Comparative Nephrotoxicity and Ototoxicity in Animal Models
Compound | Nephrotoxicity (Urinary Biomarkers)* | Ototoxicity (Cochlear Damage) |
---|---|---|
This compound | Mild (↑ lysosomal enzymes) | Lowest among aminoglycosides |
Gentamicin | Severe (↑ proteinuria, LDH) | High |
Kanamycin | Moderate | High |
Dibekacin | Severe | Moderate |
*Biomarkers include α-fucosidase, β-N-acetylglucosaminidase, and lactic dehydrogenase (LDH) .
Research Findings
Nephrotoxicity :
- In rats, ribostamycin (40 mg/kg/day for 14 days) caused minimal renal damage, while gentamicin at the same dose induced significant tubular necrosis .
- Ribostamycin accumulated in kidneys at 3× lower levels than gentamicin, correlating with reduced toxicity .
Mechanistic Uniqueness :
- This compound inhibits bacterial protein synthesis and PDI chaperone activity, a dual mechanism absent in gentamicin or kanamycin .
Spectrum of Activity :
Q & A
Basic Research Questions
Q. What are the key physicochemical properties of ribostamycin sulfate that influence experimental design in antimicrobial studies?
this compound (C₁₇H₃₄N₄O₁₀·H₂SO₄; MW 552.55, CAS 53797-35-6) is a water-soluble aminoglycoside antibiotic with limited solubility in ethanol and ether . Its hygroscopic nature necessitates storage at -20°C (powder) or -80°C (in solvent) to maintain stability . For in vitro assays, dissolve in water at 10 mg/mL, filter-sterilize (0.22 µm), and verify purity via thin-layer chromatography (TLC) or UV spectroscopy (λmax 268 nm) . Adjust pH to 6.0–7.5 to prevent degradation during long-term incubation .
Q. How should researchers validate the purity and activity of this compound batches prior to in vivo studies?
Follow pharmacopeial standards:
- Purity : Use TLC with a chloroform-methanol-ammonia (2:2:1) solvent system; RF ≈ 0.45 .
- Potency : Confirm activity via broth microdilution against reference strains (e.g., E. coli ATCC 25922), comparing MICs to literature values (e.g., 18.7 mg/L for Borrelia burgdorferi) .
- Contaminants : Test for heavy metals (<20 ppm) and sulfate ash (<0.1%) per JP Antibiotic Standards .
Advanced Research Questions
Q. What molecular interactions underlie this compound’s dual inhibition of bacterial ribosomes and protein disulfide isomerase (PDI)?
Ribostamycin binds the 30S/50S ribosomal subunits via hydrogen bonding with 16S rRNA nucleotides (e.g., A1408, G1494), disrupting translation initiation . Concurrently, it inhibits PDI’s chaperone activity (KD = 0.319 mM) by competitively blocking substrate binding at the b’ domain, requiring a 100:1 molar ratio for near-complete suppression . Use molecular docking (e.g., AutoDock Vina) with PDB 1QRG to map binding poses, prioritizing residues involved in hydrophobic interactions and hydrogen bonds (e.g., Cys53, His256) .
Q. How can researchers resolve contradictions in ototoxicity data between in vitro and in vivo models?
Discrepancies often arise from pharmacokinetic factors:
- Dosing : In guinea pigs, ribostamycin (400 mg/kg/day) achieves lower perilymph concentrations vs. gentamicin, reducing cochlear toxicity .
- Assay Design : Use ex vivo cochlear cultures with LC-MS/MS to quantify drug accumulation, paired with auditory brainstem response (ABR) testing in vivo .
- Statistical Rigor : Apply mixed-effects models to account for inter-animal variability, reporting p-values with Bonferroni correction .
Q. What methodological optimizations improve MIC assay reproducibility for ribostamycin-resistant pathogens?
- Standardization : Adopt CLSI M07-A11 guidelines, using cation-adjusted Mueller-Hinton broth and 16–20 h incubation at 35°C .
- Enzyme Inhibition : Pre-treat bacteria with β-lactamase inhibitors (e.g., clavulanic acid) to suppress aminoglycoside-modifying enzymes .
- Data Validation : Include three technical replicates per isolate and report geometric mean MICs with 95% confidence intervals .
Q. Methodological Frameworks
Q. How to design a PICOT-compliant study evaluating ribostamycin’s efficacy against multidrug-resistant Pseudomonas?
- Population : Hospital-acquired P. aeruginosa isolates (n = 50) with aminoglycoside resistance genes (e.g., aac(6')-Ib) .
- Intervention : Ribostamycin (8–64 µg/mL) ± efflux pump inhibitors (e.g., PAβN) .
- Comparison : Gentamicin and amikacin at equivalent concentrations .
- Outcome : MIC50 reduction ≥4-fold after 24 h .
- Time : 48 h post-treatment for viability assays (CFU counts) .
Q. What statistical approaches are critical for meta-analyses of ribostamycin’s nephrotoxicity across species?
- Heterogeneity Assessment : Use I² statistics to quantify variability between studies (e.g., rat vs. primate models) .
- Dose-Response Modeling : Fit log-linear regressions to serum creatinine levels vs. cumulative dose (mg/kg), adjusting for glomerular filtration rate (GFR) .
- Publication Bias : Apply Egger’s regression test to funnel plots of effect sizes .
Q. Data Presentation Guidelines
Q. How to format ribostamycin-related data for publication in compliance with CONSORT and STROBE?
- Tables : Number with Roman numerals, include footnotes for abbreviations (e.g., MIC, PDI), and align significant figures to instrument precision (e.g., 18.7 ± 1.2 mg/L) .
- Figures : Submit docking poses (e.g., PyMOL renders) as TIFF files (600 dpi), annotating hydrogen bonds and hydrophobic pockets .
- Supplemental Data : Upload raw qPCR Ct values and LC-MS/MS spectra as .csv files, citing them in the Methods .
Eigenschaften
CAS-Nummer |
53797-35-6 |
---|---|
Molekularformel |
C17H36N4O14S |
Molekulargewicht |
552.6 g/mol |
IUPAC-Name |
(2R,3S,4R,5R,6R)-5-amino-2-(aminomethyl)-6-[(1R,2R,3S,4R,6S)-4,6-diamino-2-[(3R,4S,5R)-3,4-dihydroxy-5-(hydroxymethyl)oxolan-2-yl]oxy-3-hydroxycyclohexyl]oxyoxane-3,4-diol;sulfuric acid |
InChI |
InChI=1S/C17H34N4O10.H2O4S/c18-2-6-10(24)12(26)8(21)16(28-6)30-14-5(20)1-4(19)9(23)15(14)31-17-13(27)11(25)7(3-22)29-17;1-5(2,3)4/h4-17,22-27H,1-3,18-21H2;(H2,1,2,3,4)/t4-,5+,6-,7-,8-,9+,10-,11-,12-,13-,14-,15-,16-,17?;/m1./s1 |
InChI-Schlüssel |
RTCDDYYZMGGHOE-CZUQXGEQSA-N |
SMILES |
C1C(C(C(C(C1N)OC2C(C(C(C(O2)CN)O)O)N)OC3C(C(C(O3)CO)O)O)O)N.[O-]S(=O)(=O)[O-] |
Isomerische SMILES |
C1[C@H]([C@@H]([C@H]([C@@H]([C@H]1N)O[C@@H]2[C@@H]([C@H]([C@@H]([C@H](O2)CN)O)O)N)OC3[C@@H]([C@@H]([C@H](O3)CO)O)O)O)N.OS(=O)(=O)O |
Kanonische SMILES |
C1C(C(C(C(C1N)OC2C(C(C(C(O2)CN)O)O)N)OC3C(C(C(O3)CO)O)O)O)N.OS(=O)(=O)O |
Key on ui other cas no. |
53797-35-6 |
Piktogramme |
Irritant; Health Hazard |
Synonyme |
Vistamycin Sulfate |
Herkunft des Produkts |
United States |
Retrosynthesis Analysis
AI-Powered Synthesis Planning: Our tool employs the Template_relevance Pistachio, Template_relevance Bkms_metabolic, Template_relevance Pistachio_ringbreaker, Template_relevance Reaxys, Template_relevance Reaxys_biocatalysis model, leveraging a vast database of chemical reactions to predict feasible synthetic routes.
One-Step Synthesis Focus: Specifically designed for one-step synthesis, it provides concise and direct routes for your target compounds, streamlining the synthesis process.
Accurate Predictions: Utilizing the extensive PISTACHIO, BKMS_METABOLIC, PISTACHIO_RINGBREAKER, REAXYS, REAXYS_BIOCATALYSIS database, our tool offers high-accuracy predictions, reflecting the latest in chemical research and data.
Strategy Settings
Precursor scoring | Relevance Heuristic |
---|---|
Min. plausibility | 0.01 |
Model | Template_relevance |
Template Set | Pistachio/Bkms_metabolic/Pistachio_ringbreaker/Reaxys/Reaxys_biocatalysis |
Top-N result to add to graph | 6 |
Feasible Synthetic Routes
Haftungsausschluss und Informationen zu In-Vitro-Forschungsprodukten
Bitte beachten Sie, dass alle Artikel und Produktinformationen, die auf BenchChem präsentiert werden, ausschließlich zu Informationszwecken bestimmt sind. Die auf BenchChem zum Kauf angebotenen Produkte sind speziell für In-vitro-Studien konzipiert, die außerhalb lebender Organismen durchgeführt werden. In-vitro-Studien, abgeleitet von dem lateinischen Begriff "in Glas", beinhalten Experimente, die in kontrollierten Laborumgebungen unter Verwendung von Zellen oder Geweben durchgeführt werden. Es ist wichtig zu beachten, dass diese Produkte nicht als Arzneimittel oder Medikamente eingestuft sind und keine Zulassung der FDA für die Vorbeugung, Behandlung oder Heilung von medizinischen Zuständen, Beschwerden oder Krankheiten erhalten haben. Wir müssen betonen, dass jede Form der körperlichen Einführung dieser Produkte in Menschen oder Tiere gesetzlich strikt untersagt ist. Es ist unerlässlich, sich an diese Richtlinien zu halten, um die Einhaltung rechtlicher und ethischer Standards in Forschung und Experiment zu gewährleisten.