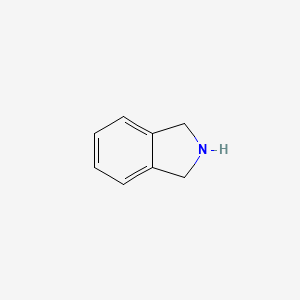
Isoindolin
Übersicht
Beschreibung
2,3-Dihydro-1H-isoindole is an organic compound with the molecular formula C8H9N. It is a heterocyclic compound featuring a nitrogen atom within a six-membered ring structure.
Wissenschaftliche Forschungsanwendungen
2,3-Dihydro-1H-isoindol hat eine breite Palette von Anwendungen in der wissenschaftlichen Forschung:
5. Wirkmechanismus
Der Wirkmechanismus von 2,3-Dihydro-1H-isoindol beinhaltet seine Interaktion mit verschiedenen molekularen Zielen und Signalwegen. Das aromatische Ringsystem der Verbindung ermöglicht es ihr, mit hoher Affinität an mehrere Rezeptoren zu binden und beeinflusst so biologische Prozesse wie Enzyminhibition und Rezeptormodulation . Das Vorhandensein des Stickstoffatoms ermöglicht es der Verbindung auch, an Wasserstoffbrückenbindungen und anderen intermolekularen Wechselwirkungen teilzunehmen, was ihre biologische Aktivität weiter erhöht .
Ähnliche Verbindungen:
Indol: Eine strukturell verwandte Verbindung mit einem Benzolring, der mit einem Pyrrolring verschmolzen ist.
2,3-Dihydroindol: Eine Verbindung mit einer ähnlichen Struktur, die sich jedoch in der Position des Stickstoffatoms und im Vorhandensein zusätzlicher funktioneller Gruppen unterscheidet.
Einzigartigkeit: 2,3-Dihydro-1H-isoindol ist aufgrund seiner spezifischen Ringstruktur und dem Vorhandensein des Stickstoffatoms einzigartig, was ihm eine besondere chemische Reaktivität und biologische Aktivität verleiht.
Safety and Hazards
Zukünftige Richtungen
Isoindolines are the focus of much research because of being an important family of compounds present in a wide array of bioactive molecules . The development of new derivatives of isoindoline-1,3-dione is relevant . The aim is to develop a green synthesis technique for isoindolines/dioxoisoindolines .
Wirkmechanismus
Target of Action
Isoindoline and its derivatives have been found to interact with various biological targets. For instance, isoindoline-1,3-dione derivatives have shown potential in modulating the dopamine receptor D3 , suggesting a potential application as antipsychotic agents. Additionally, isoindoline-1-ones have been explored as potential inhibitors of Cyclin-dependent kinases (CDKs) , which are crucial for cell cycle regulation. Furthermore, isoindoline derivatives have been evaluated for their inhibitory effects on cholinesterases , enzymes essential for neurotransmission.
Mode of Action
The interaction of isoindoline with its targets involves binding at specific sites, leading to changes in the target’s function. For instance, isoindoline-1-ones have shown high binding affinity and conventional hydrogen bonding interactions with active amino acid residues of CDK7 . This interaction can inhibit the kinase activity of CDK7, thereby affecting cell cycle progression.
Biochemical Pathways
Isoindoline’s interaction with its targets can affect various biochemical pathways. For instance, by inhibiting CDKs, isoindoline-1-ones can disrupt the cell cycle, potentially leading to cell cycle arrest . Moreover, by modulating the activity of cholinesterases, isoindoline derivatives can influence neurotransmission .
Pharmacokinetics
The ADME (Absorption, Distribution, Metabolism, and Excretion) properties of isoindoline derivatives have been studied. For instance, isoindoline amide derivatives have shown excellent drug-like properties and better pharmacokinetic profiles than some known CDK7 inhibitors . These properties can significantly impact the bioavailability of the compound, influencing its therapeutic efficacy.
Result of Action
The molecular and cellular effects of isoindoline’s action depend on its specific targets. For instance, by inhibiting CDKs, isoindoline-1-ones can induce cell cycle arrest, potentially leading to apoptosis . Moreover, modulation of cholinesterase activity can affect neurotransmission, potentially influencing cognitive function .
Action Environment
The action, efficacy, and stability of isoindoline can be influenced by various environmental factors. For instance, the synthesis and reactivity of isoindoline-1,3-dione derivatives can be influenced by the specific substituents attached to both the isoindoline core and the carbonyl groups . Understanding these influences is crucial for optimizing the therapeutic use of isoindoline and its derivatives.
Biochemische Analyse
Biochemical Properties
Isoindoline plays a crucial role in biochemical reactions, particularly in the context of enzyme interactions. It has been observed to interact with enzymes such as O-GlcNAcase and O-GlcNAc transferase, which are involved in the post-translational modification of proteins by adding or removing N-acetylglucosamine residues . These interactions are essential for regulating various biological processes, including cell signaling and gene expression. Isoindoline’s ability to modulate these enzymes highlights its potential as a therapeutic agent in diseases where these pathways are dysregulated.
Cellular Effects
Isoindoline influences various cellular processes, including cell signaling pathways, gene expression, and cellular metabolism. It has been shown to affect the O-GlcNAcylation process, which is a post-translational modification that impacts protein function and stability . By modulating the activity of O-GlcNAcase and O-GlcNAc transferase, Isoindoline can alter the levels of O-GlcNAc-modified proteins, thereby influencing cell function and signaling pathways. This modulation can have significant effects on cellular processes such as proliferation, differentiation, and apoptosis.
Molecular Mechanism
The molecular mechanism of Isoindoline involves its interaction with specific enzymes and proteins. Isoindoline binds to the active site of O-GlcNAcase, inhibiting its activity and preventing the removal of N-acetylglucosamine residues from proteins . This inhibition leads to an accumulation of O-GlcNAc-modified proteins, which can alter cellular signaling and gene expression. Additionally, Isoindoline can interact with other biomolecules, such as transcription factors, to modulate their activity and influence gene expression patterns.
Temporal Effects in Laboratory Settings
In laboratory settings, the effects of Isoindoline have been observed to change over time. Isoindoline is relatively stable under standard laboratory conditions, but it can degrade over extended periods, leading to a decrease in its efficacy . Long-term studies have shown that Isoindoline can have sustained effects on cellular function, particularly in terms of altering gene expression and protein modification. The stability and degradation of Isoindoline must be carefully monitored to ensure consistent results in experimental studies.
Dosage Effects in Animal Models
The effects of Isoindoline vary with different dosages in animal models. At low doses, Isoindoline has been shown to modulate enzyme activity and protein modification without causing significant toxicity . At higher doses, Isoindoline can induce adverse effects, including cytotoxicity and disruption of normal cellular processes. Threshold effects have been observed, where a specific dosage range is required to achieve the desired biochemical effects without causing harm to the organism.
Metabolic Pathways
Isoindoline is involved in various metabolic pathways, particularly those related to protein modification and degradation. It interacts with enzymes such as O-GlcNAcase and O-GlcNAc transferase, which play key roles in the O-GlcNAcylation process . Isoindoline’s impact on these enzymes can influence metabolic flux and alter the levels of specific metabolites within the cell. Additionally, Isoindoline can affect other metabolic pathways by modulating the activity of enzymes involved in protein synthesis and degradation.
Transport and Distribution
Within cells and tissues, Isoindoline is transported and distributed through interactions with specific transporters and binding proteins. These interactions facilitate the localization and accumulation of Isoindoline in specific cellular compartments . The distribution of Isoindoline can influence its activity and efficacy, as it needs to reach its target enzymes and proteins to exert its biochemical effects. Understanding the transport and distribution mechanisms of Isoindoline is crucial for optimizing its therapeutic potential.
Subcellular Localization
Isoindoline’s subcellular localization is influenced by targeting signals and post-translational modifications that direct it to specific compartments or organelles. Isoindoline has been observed to localize in the cytoplasm and nucleus, where it interacts with enzymes and proteins involved in the O-GlcNAcylation process . This localization is essential for its activity, as it needs to be in proximity to its target biomolecules to exert its effects. Additionally, post-translational modifications of Isoindoline can further influence its localization and function within the cell.
Vorbereitungsmethoden
Synthetic Routes and Reaction Conditions: One common method for synthesizing 2,3-Dihydro-1H-isoindole involves the reaction of aniline with α, β-unsaturated ketones, such as indoline-2,3-diketone. This reaction typically undergoes oxidation, reduction, and amidation processes to yield the target product . Another efficient method involves the use of methanesulfonic acid under reflux conditions in methanol, which provides a good yield of the desired compound .
Industrial Production Methods: Industrial production of 2,3-Dihydro-1H-isoindole often employs large-scale chemical synthesis techniques, utilizing readily available starting materials and optimized reaction conditions to maximize yield and purity. The use of continuous flow reactors and advanced purification methods, such as preparative thin-layer chromatography, are common in industrial settings .
Analyse Chemischer Reaktionen
Reaktionstypen: 2,3-Dihydro-1H-isoindol durchläuft verschiedene chemische Reaktionen, darunter Oxidation, Reduktion und Substitution. Diese Reaktionen werden durch das Vorhandensein des Stickstoffatoms und des konjugierten Ringsystems erleichtert, die Angriffspunkte für elektrophile und nukleophile Angriffe bieten .
Häufige Reagenzien und Bedingungen:
Hauptprodukte, die gebildet werden: Die Hauptprodukte, die aus diesen Reaktionen gebildet werden, umfassen verschiedene substituierte Isoindole und Indole, die je nach Art der Substituenten unterschiedliche chemische und biologische Eigenschaften aufweisen können .
Vergleich Mit ähnlichen Verbindungen
Indole: A structurally related compound with a benzene ring fused to a pyrrole ring.
2,3-Dihydroindole: A compound with a similar structure but differing in the position of the nitrogen atom and the presence of additional functional groups.
Uniqueness: 2,3-Dihydro-1H-isoindole is unique due to its specific ring structure and the presence of the nitrogen atom, which confer distinct chemical reactivity and biological activity.
Eigenschaften
IUPAC Name |
2,3-dihydro-1H-isoindole | |
---|---|---|
Source | PubChem | |
URL | https://pubchem.ncbi.nlm.nih.gov | |
Description | Data deposited in or computed by PubChem | |
InChI |
InChI=1S/C8H9N/c1-2-4-8-6-9-5-7(8)3-1/h1-4,9H,5-6H2 | |
Source | PubChem | |
URL | https://pubchem.ncbi.nlm.nih.gov | |
Description | Data deposited in or computed by PubChem | |
InChI Key |
GWVMLCQWXVFZCN-UHFFFAOYSA-N | |
Source | PubChem | |
URL | https://pubchem.ncbi.nlm.nih.gov | |
Description | Data deposited in or computed by PubChem | |
Canonical SMILES |
C1C2=CC=CC=C2CN1 | |
Source | PubChem | |
URL | https://pubchem.ncbi.nlm.nih.gov | |
Description | Data deposited in or computed by PubChem | |
Molecular Formula |
C8H9N | |
Source | PubChem | |
URL | https://pubchem.ncbi.nlm.nih.gov | |
Description | Data deposited in or computed by PubChem | |
DSSTOX Substance ID |
DTXSID20964291 | |
Record name | 2,3-Dihydro-1H-isoindole | |
Source | EPA DSSTox | |
URL | https://comptox.epa.gov/dashboard/DTXSID20964291 | |
Description | DSSTox provides a high quality public chemistry resource for supporting improved predictive toxicology. | |
Molecular Weight |
119.16 g/mol | |
Source | PubChem | |
URL | https://pubchem.ncbi.nlm.nih.gov | |
Description | Data deposited in or computed by PubChem | |
CAS No. |
496-12-8 | |
Record name | Isoindoline | |
Source | CAS Common Chemistry | |
URL | https://commonchemistry.cas.org/detail?cas_rn=496-12-8 | |
Description | CAS Common Chemistry is an open community resource for accessing chemical information. Nearly 500,000 chemical substances from CAS REGISTRY cover areas of community interest, including common and frequently regulated chemicals, and those relevant to high school and undergraduate chemistry classes. This chemical information, curated by our expert scientists, is provided in alignment with our mission as a division of the American Chemical Society. | |
Explanation | The data from CAS Common Chemistry is provided under a CC-BY-NC 4.0 license, unless otherwise stated. | |
Record name | 2,3-Dihydro-1H-isoindole | |
Source | EPA DSSTox | |
URL | https://comptox.epa.gov/dashboard/DTXSID20964291 | |
Description | DSSTox provides a high quality public chemistry resource for supporting improved predictive toxicology. | |
Record name | Isoindoline | |
Source | European Chemicals Agency (ECHA) | |
URL | https://echa.europa.eu/information-on-chemicals | |
Description | The European Chemicals Agency (ECHA) is an agency of the European Union which is the driving force among regulatory authorities in implementing the EU's groundbreaking chemicals legislation for the benefit of human health and the environment as well as for innovation and competitiveness. | |
Explanation | Use of the information, documents and data from the ECHA website is subject to the terms and conditions of this Legal Notice, and subject to other binding limitations provided for under applicable law, the information, documents and data made available on the ECHA website may be reproduced, distributed and/or used, totally or in part, for non-commercial purposes provided that ECHA is acknowledged as the source: "Source: European Chemicals Agency, http://echa.europa.eu/". Such acknowledgement must be included in each copy of the material. ECHA permits and encourages organisations and individuals to create links to the ECHA website under the following cumulative conditions: Links can only be made to webpages that provide a link to the Legal Notice page. | |
Record name | Isoindoline | |
Source | FDA Global Substance Registration System (GSRS) | |
URL | https://gsrs.ncats.nih.gov/ginas/app/beta/substances/4G7B55YCK6 | |
Description | The FDA Global Substance Registration System (GSRS) enables the efficient and accurate exchange of information on what substances are in regulated products. Instead of relying on names, which vary across regulatory domains, countries, and regions, the GSRS knowledge base makes it possible for substances to be defined by standardized, scientific descriptions. | |
Explanation | Unless otherwise noted, the contents of the FDA website (www.fda.gov), both text and graphics, are not copyrighted. They are in the public domain and may be republished, reprinted and otherwise used freely by anyone without the need to obtain permission from FDA. Credit to the U.S. Food and Drug Administration as the source is appreciated but not required. | |
Synthesis routes and methods I
Procedure details
Synthesis routes and methods II
Procedure details
Synthesis routes and methods III
Procedure details
Synthesis routes and methods IV
Procedure details
Retrosynthesis Analysis
AI-Powered Synthesis Planning: Our tool employs the Template_relevance Pistachio, Template_relevance Bkms_metabolic, Template_relevance Pistachio_ringbreaker, Template_relevance Reaxys, Template_relevance Reaxys_biocatalysis model, leveraging a vast database of chemical reactions to predict feasible synthetic routes.
One-Step Synthesis Focus: Specifically designed for one-step synthesis, it provides concise and direct routes for your target compounds, streamlining the synthesis process.
Accurate Predictions: Utilizing the extensive PISTACHIO, BKMS_METABOLIC, PISTACHIO_RINGBREAKER, REAXYS, REAXYS_BIOCATALYSIS database, our tool offers high-accuracy predictions, reflecting the latest in chemical research and data.
Strategy Settings
Precursor scoring | Relevance Heuristic |
---|---|
Min. plausibility | 0.01 |
Model | Template_relevance |
Template Set | Pistachio/Bkms_metabolic/Pistachio_ringbreaker/Reaxys/Reaxys_biocatalysis |
Top-N result to add to graph | 6 |
Feasible Synthetic Routes
Q1: What are some common methods for synthesizing isoindoline derivatives?
A1: Several synthetic approaches are available for isoindoline derivatives:
- Condensation Reactions:
- Reacting barbituric acid with 1,3-diimino isoindoline in an inorganic acid-methanol solution yields isoindoline yellow pigments [].
- Condensing phthalic anhydride with various anilines in the presence of Water Extract of Onion Peel Ash (WEOPA) as a green catalyst provides access to isoindoline-1,3-dione derivatives [].
- Alkylation and Reduction:
- Cyclocondensation:
- Palladium-Catalyzed Cascade Reactions:
Q2: What is the significance of using WEOPA as a catalyst in isoindoline synthesis?
A2: WEOPA offers several advantages as a green catalytic system for synthesizing isoindoline-1,3-dione derivatives []:
Q3: How is the structure of 2-(3-(4-phenylpiperazin-1-yl)propyl)isoindoline-1,3-dione characterized?
A3: This compound is characterized using a combination of techniques:
- NMR Spectroscopy: Proton and carbon NMR (1H-NMR and 13C-NMR) provide information about the compound's hydrogen and carbon framework [].
- Single Crystal X-Ray Diffraction: This method reveals the compound's three-dimensional structure, including bond lengths, angles, and molecular packing in the crystal lattice [].
Q4: How do spectroscopic techniques aid in characterizing isoindoline derivatives?
A4: Various spectroscopic methods are employed for structural elucidation:
- IR Spectroscopy: Identifies functional groups present in the molecule based on their characteristic vibrational frequencies [, , ].
- Mass Spectrometry (MS): Determines the molecular weight and fragmentation pattern, providing insights into the compound's structure [].
- NMR Spectroscopy: Provides detailed information about the arrangement of carbon and hydrogen atoms in the molecule [, , ].
- X-Ray Photoelectron Spectroscopy (XPS): Used to analyze the elemental composition and chemical states within a material, particularly useful for studying nitrogen-containing functional groups like nitroxides [].
Q5: How does density functional theory (DFT) contribute to understanding isoindoline derivatives?
A5: DFT calculations offer valuable insights into the electronic and structural properties of isoindolines:
- Electronic Structure: DFT helps determine the highest occupied molecular orbital (HOMO) and lowest unoccupied molecular orbital (LUMO) energies, providing insights into electronic transitions, redox properties, and potential applications in organic electronics [, ].
- Molecular Geometry: DFT optimizes the molecular geometry of isoindoline derivatives, allowing researchers to study bond lengths, angles, and overall molecular shape [, ].
- Optical Properties: DFT calculations help predict the UV-visible absorption and emission spectra of isoindoline-based dyes, providing information about their color and potential applications in optoelectronic devices [].
Q6: What are some notable applications of isoindoline derivatives?
A6: Isoindolines exhibit a range of applications, including:
- Pigments: Isoindoline-based compounds are used as pigments in various applications, including inks, paints, and plastics [, ].
- Antidepressants: Certain isoindoline derivatives have shown promising antidepressant activity in preclinical studies [].
- Antimicrobial Agents: Some isoindoline derivatives exhibit antimicrobial activity against bacteria and fungi [, ].
- Spin Probes: Isoindoline nitroxides, due to their paramagnetic nature, serve as spin probes in electron paramagnetic resonance (EPR) spectroscopy, enabling the study of molecular dynamics and interactions in biological systems [, , , , ].
- Fluorescence-Suppressed Probes: Isoindoline nitroxide-labeled porphyrins act as fluorescence-suppressed probes, where fluorescence is quenched by the nitroxide and restored upon radical scavenging, allowing for monitoring polymer degradation [].
- Enzyme Inhibitors: Isoindoline derivatives have been explored as inhibitors of enzymes like cyclooxygenase (COX), which are involved in inflammation, and 4-hydroxyphenylpyruvate dioxygenase (HPPD), a target for herbicide development [, ].
Q7: How do isoindoline derivatives exert their antidepressant effects?
A7: While the exact mechanism is still under investigation, research suggests that isoindoline derivatives may exert antidepressant effects by increasing serotonin (5-HT) levels in the brain [].
Q8: How do the structures of isoindoline-1,3-dione derivatives relate to their COX inhibitory activity?
A8: The presence and position of substituents on the isoindoline-1,3-dione core influence their interactions with COX enzymes and thus affect their inhibitory activity. For instance, compounds with a bulky aryl group tend to exhibit higher potency toward COX-2, whereas those with a more accessible amino group might display selectivity for COX-1 [, ].
Q9: How are isoindoline nitroxides utilized in studying polymer degradation?
A9: Isoindoline nitroxides, either alone or linked to fluorophores, act as sensitive probes for monitoring polymer degradation:
- Direct Monitoring: The decrease in the EPR signal of the nitroxide, due to its reaction with radicals formed during polymer degradation, provides a direct measure of degradation [, ].
- Profluorescent Nitroxides: When linked to a fluorophore, the fluorescence of the system is initially quenched by the nitroxide. As degradation occurs and the nitroxide scavenges radicals, fluorescence increases, enabling visualization and quantification of degradation processes [].
Q10: How do isoindoline derivatives interact with DNA?
A10: Isoindoline derivatives exhibit diverse DNA binding modes depending on their structure:
- Groove Binding: Some derivatives bind to the grooves of the DNA helix [].
- Intercalation: Certain compounds, particularly those with planar aromatic systems, can intercalate between DNA base pairs [].
- Abasic Site Binding: Nitroxide-derived N-oxide phenazines containing isoindoline moieties have shown selective binding affinity for abasic sites in duplex DNA, particularly those with cytosine as the orphan base [].
Q11: What are the implications of inhibiting the BfrB-Bfd interaction in Pseudomonas aeruginosa?
A12: Inhibiting the interaction between the bacterial iron storage protein bacterioferritin (BfrB) and its ferredoxin partner (Bfd) disrupts iron homeostasis in P. aeruginosa []. This leads to:
Q12: How does the structure of bis(2-pyridylimino)isoindoline derivatives impact their birefringence?
A13: The birefringence (Δn) of bis(2-pyridylimino)isoindoline crystals is significantly influenced by the substituents attached to the isoindoline core. Bulky substituents and their orientation in the crystal lattice affect the anisotropy of molecular polarizability, leading to variations in birefringence values [].
Q13: How does the choice of solvent affect the properties of isoindoline derivatives?
A14: Theoretical calculations on isoindoline derivatives indicate that solvent polarity can influence their electronic properties and tautomeric equilibria. For instance, isoindoline tautomers are generally more stable in polar solvents like DMSO compared to less polar solvents like THF [].
Q14: How can halogen bonding be utilized in designing self-assembling organic spin systems based on isoindoline nitroxides?
A15: Halogen bonding, a noncovalent interaction between an electron-deficient halogen atom and an electron-rich atom, can direct the self-assembly of isoindoline nitroxide molecules. For example, the interaction between 1,1,3,3-tetramethylisoindolin-2-yloxyl (TMIO) and 1,4-diiodotetrafluorobenzene leads to the formation of a discrete 2:1 supramolecular complex stabilized by N—O···I halogen bonds. This highlights the potential of using halogen bonding for creating ordered architectures of paramagnetic molecules [].
Haftungsausschluss und Informationen zu In-Vitro-Forschungsprodukten
Bitte beachten Sie, dass alle Artikel und Produktinformationen, die auf BenchChem präsentiert werden, ausschließlich zu Informationszwecken bestimmt sind. Die auf BenchChem zum Kauf angebotenen Produkte sind speziell für In-vitro-Studien konzipiert, die außerhalb lebender Organismen durchgeführt werden. In-vitro-Studien, abgeleitet von dem lateinischen Begriff "in Glas", beinhalten Experimente, die in kontrollierten Laborumgebungen unter Verwendung von Zellen oder Geweben durchgeführt werden. Es ist wichtig zu beachten, dass diese Produkte nicht als Arzneimittel oder Medikamente eingestuft sind und keine Zulassung der FDA für die Vorbeugung, Behandlung oder Heilung von medizinischen Zuständen, Beschwerden oder Krankheiten erhalten haben. Wir müssen betonen, dass jede Form der körperlichen Einführung dieser Produkte in Menschen oder Tiere gesetzlich strikt untersagt ist. Es ist unerlässlich, sich an diese Richtlinien zu halten, um die Einhaltung rechtlicher und ethischer Standards in Forschung und Experiment zu gewährleisten.