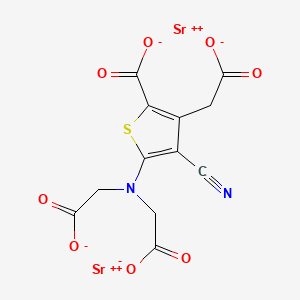
Strontium ranelate
Overview
Description
Strontium ranelate is a medication primarily used for the treatment of severe osteoporosis in postmenopausal women and adult men at high risk of fractures . It is a strontium (II) salt of ranelic acid and is known for its unique dual action on bone metabolism, both increasing bone formation by osteoblasts and reducing bone resorption by osteoclasts .
Scientific Research Applications
Strontium ranelate has a wide range of scientific research applications:
Chemistry: Used as a model compound to study the effects of strontium on bone mineral density and structure.
Biology: Investigated for its role in bone metabolism and its potential effects on other biological systems.
Industry: Utilized in the development of new bone-strengthening agents and materials.
Mechanism of Action
Target of Action
Strontium ranelate primarily targets osteoblasts and osteoclasts , the two key cell types involved in bone remodeling . Osteoblasts are responsible for bone formation, while osteoclasts are involved in bone resorption .
Mode of Action
This compound has a unique mode of action. It increases the deposition of new bone by stimulating osteoblasts and simultaneously reduces the resorption of bone by inhibiting osteoclasts . This dual action on both osteoblasts and osteoclasts is why it’s often referred to as a “dual action bone agent” (DABA) .
Biochemical Pathways
This compound influences several biochemical pathways. It activates pre-osteoblast replication, osteoblast differentiation, and survival, and reduces pre-osteoclast differentiation, osteoclast function, and survival . These effects are mediated at least in part via the calcium-sensing receptor (CaSR) , nuclear factor of activated Tc (NFATc)/Wnt signaling , and modulation of the osteoprotegerin (OPG)/RANKL ratio .
Pharmacokinetics
This compound is administered orally. It has a high affinity for bone tissue and is excreted through renal and gastrointestinal routes . The elimination half-life is approximately 60 hours .
Result of Action
The action of this compound results in improved bone microarchitecture and increased bone mineral density . It enhances the replication of preosteoblastic cells, stimulates the synthesis of collagen and noncollagenic proteins by osteoblasts , and decreases bone resorption . This leads to an increase in bone mass and a decrease in the risk of fractures .
Action Environment
The efficacy of this compound can be influenced by various environmental factors. For instance, the form of strontium used can affect its impact on bone structure . Additionally, factors such as age, body mass index, smoking habits, and other health conditions can influence the drug’s efficacy and safety .
Safety and Hazards
Biochemical Analysis
Biochemical Properties
Strontium ranelate activates pre-osteoblast replication, osteoblast differentiation and survival, and reduces pre-osteoclast differentiation, osteoclast function, and survival . It acts at least in part via the calcium-sensing receptor (CaSR), nuclear factor of activated Tc (NFATc)/Wnt signaling, and modulation of the osteoprotegerin (OPG)/RANKL ratio .
Cellular Effects
This compound has been shown to have a significant impact on various types of cells and cellular processes. It increases the replication of pre-osteoblastic cells and enhances the synthesis of collagen and noncollagenic proteins by osteoblasts . It also inhibits osteoclast differentiation and activity . These effects result in an increase in bone mass and improvements in the micro-architecture of bone tissue .
Molecular Mechanism
The molecular mechanism of this compound involves its interaction with the calcium-sensing receptor (CaSR). This interaction leads to the activation of pre-osteoblast replication, osteoblast differentiation, and survival, and the reduction of pre-osteoclast differentiation, osteoclast function, and survival . This dual action on bone formation and resorption is unique to this compound and is key to its efficacy in treating osteoporosis .
Temporal Effects in Laboratory Settings
In a 2-year study, this compound demonstrated a dose-dependent increase in bone strength and bone mass of the vertebral body and midshaft femur without change in bone stiffness . This increase in mechanical properties was associated with improvements in the micro-architecture of bone tissue .
Dosage Effects in Animal Models
In animal models, this compound has been shown to increase bone strength and bone mass in a dose-dependent manner . It has also been shown to reduce the number of osteoclasts . These effects were observed with long-term treatment using high doses .
Metabolic Pathways
This compound is involved in the metabolic pathways that regulate bone formation and resorption . It acts through the calcium-sensing receptor (CaSR) in bone tissue cells . This interaction leads to the activation of pre-osteoblast replication and osteoblast differentiation, and the reduction of pre-osteoclast differentiation and osteoclast function .
Transport and Distribution
Strontium is distributed in calcified matrix and is easily exchangeable from bone mineral, being slightly linked to mature crystals through ionic substitutions . The heterogeneity of strontium distribution in bone and the quality of bone mineral crystals formed during this compound administration may explain its beneficial effects on reducing the risk of fractures .
Subcellular Localization
The subcellular localization of this compound is primarily within the calcified matrix of bone tissue . It is easily exchangeable from bone mineral and is slightly linked to mature crystals through ionic substitutions . This localization is key to its role in increasing bone mass and reducing the risk of fractures .
Preparation Methods
Synthetic Routes and Reaction Conditions: The preparation of high-purity strontium ranelate involves several steps:
Reaction of acetonedicarboxylic acid diethyl ester with malononitrile: This reaction occurs in the presence of an acid-binding agent and ethanol to generate an active intermediate.
Cyclization reaction: The active intermediate undergoes cyclization with sulfur under reflux of ethanol to form 5-amino-4-cyano-3-(2-ethyoxyl-2-carboxymethyl)-thiophene-2-ethyl formate (Intermediate I).
Hydrocarbonylation: Intermediate I reacts with a hydrocarbonylation reagent in the presence of potassium carbonate and a catalyst to produce tetraethyl ranelate.
Industrial Production Methods: Industrial production methods for this compound typically follow the same synthetic routes but are optimized for large-scale production. This includes the use of high-efficiency reactors, continuous processing techniques, and stringent quality control measures to ensure high purity and stability of the final product .
Chemical Reactions Analysis
Types of Reactions: Strontium ranelate undergoes various chemical reactions, including:
Hydrolysis: The hydrolysis of tetraethyl ranelate to form this compound.
Complexation: Formation of complexes with other ions or molecules.
Common Reagents and Conditions:
Hydrolysis: Alkali metal hydroxide solutions (e.g., sodium hydroxide or potassium hydroxide) are commonly used.
Cyclization: Sulfur and ethanol under reflux conditions.
Major Products:
Comparison with Similar Compounds
Strontium Citrate: Composed of strontium and citric acid, it is another form of strontium used for bone health.
Strontium Chloride: Composed of strontium and chloride ions, it is used in various applications, including bone health supplements.
Comparison:
Bioavailability: Strontium ranelate has a higher bioavailability compared to other strontium salts, making it more effective in reaching systemic circulation.
Mechanism of Action: Unlike other strontium compounds, this compound has a unique dual action on bone metabolism, both promoting bone formation and inhibiting bone resorption.
This compound stands out due to its dual mechanism of action and higher bioavailability, making it a preferred choice for the treatment of severe osteoporosis.
Properties
{ "Design of the Synthesis Pathway": "The synthesis pathway of Strontium ranelate involves the reaction between strontium carbonate and ranelic acid.", "Starting Materials": [ "Strontium carbonate", "Ranelic acid" ], "Reaction": [ "Dissolve strontium carbonate in water to form strontium hydroxide", "React strontium hydroxide with ranelic acid to form strontium ranelate", "Filter and dry the product" ] } | |
The underlying pathogenesis of osteperosis involves an imbalance between bone resorption and bone formation. Osteoclasts are a kind of differentiated or specialized bone cell that breaks down bone tissue while osteoblasts are another set of differentiated bone cells that synthesize and rebuild bone tissue. When osteoclasts degrade bone tissue faster than the osteoblasts are capable of rebuilding the bone tissue, low or inadequate bone mass density and osteoperosis can resula One of the mechanisms with which strontium ranelate is thought to act is its functionality as an agonist of the extracellular calcium sensing receptors (CaSRs) of osteoblasts and osteoclasts. Ordinary interaction between calcium 2+ divalent cations with mature osteoclast CaSRs is known to induce osteoclast apoptosis. Subsequently, strontium 2+ divalent cations from strontium ranelate use can also bind CaSRs on osteoclasts to induce their apoptosis because of the strontium 2+ cation's close resemeblance to calcium 2+. Contact between extracelluar calcium 2+ and osteoclast CaSRs stimulates the phospholipase C (PLC) dependant breakdown of phosphatidylinositol 4,5-biphosphate (PIP2) into the two secondary messengers inositol 1,4,5-trisphosphate (IP3) and diacylglycerol (DAG). Whereas the calcium-CaSRs interaction then performs IP3 Adependent translocation of nuclear factor NF-kB from the cytoplasm to the nucleus in mature osteoclasts, strontium-CaSRs interactions involves a DAG-PKC beta II (protein kinase C beta II) signalling pathway for translocating NF-kB from the cytoplasm to the nucleus in an IP3-independent manner. Although the calcium 2+ and strontium 2+ mediated signalling pathways are different, both CaSR interactions induce osteoclast apoptosis and are in fact capable of potentiating each other, leading to enhanced osteoclast apoptosis and decreased bone tissue degradation. At the same time, given the similarity between the calcium 2+ and strontium 2+ cations, strontium 2+ cations from strontium ranelate are seemingly also able to act as an agonist and stimulate the CaSRs on osteoblasts, possibly in tandem with various local osteoblast stimulatory growth factors like transforming growth factor β (TGF β) and/or bone morphogenetic proteins (BMPs), to stimulate cyclic D genes and early oncogenes like c-fos and egr-1 that can mediate the mitogenesis and proliferation of new or more osteoblasts. Moreover, although the involvement of the PLC mediated pathway may be a part of the signalling mechanism in osteoblasts following the stimulation of their CaSRs, this has not yet been fully elucidated. Furthermore, strontium ranelate is also thought to be capable of stimulating osteoblasts to enhance the expression of osteoprotegerin while also concurrently reducing the expression of receptor activator of nuclear factor kappa-Β ligand (RANKL) in primary human osteoblastic cells. As osteoprotegerin can competitively bind to RANKL as a decoy receptor, which can prevent RANKL from binding to RANK, which is an activity that facilitates the signaling pathway for the differentiation and activaiton of osteoclasts. The subsequent net effect of these actions ultiamtely results in decreased osteoclastogenesis. Moreover, bone biopsies obtained from patients treated with stronatium ranelate in clinical study reveal improvements in intrinsic bone tissue quality and microarchitecutre in ostepoerosis as evidenced by increased trabecular number, decreased trabecular separation, lower structure model index, and increased cortical thickness associated with a shift in trabecular structure from rod to plate like configurations compared with control patients. Additionally, strontium from administered strontium ranelate is absorbed onto the crystal surface of treated bones and only slightly substitiutes for calcium in the apatite crystal of newly formed bone. As a result, there is an increased X-ray absorption of strontium as compared to calcium, which can lead to an amplification of bone mineral density (BMD) measurement by dual-proton X-ray absorptiometry. In essence, although strontium ranelate use can increase BMD some of the observations may be overestimations due to skeletal accretion of strontium in strontium ranelate treated patients. Having the ability to both generate more osetoblasts and decrease the number of osteoclasts gives strontium ranelate an apparent dual mechanism of action when used to treat osteoperosis. | |
CAS No. |
135459-87-9 |
Molecular Formula |
C12H10N2O8SSr |
Molecular Weight |
429.9 g/mol |
IUPAC Name |
distrontium;5-[bis(carboxylatomethyl)amino]-3-(carboxylatomethyl)-4-cyanothiophene-2-carboxylate |
InChI |
InChI=1S/C12H10N2O8S.Sr/c13-2-6-5(1-7(15)16)10(12(21)22)23-11(6)14(3-8(17)18)4-9(19)20;/h1,3-4H2,(H,15,16)(H,17,18)(H,19,20)(H,21,22); |
InChI Key |
SKKLLKBVXKVYLE-UHFFFAOYSA-N |
SMILES |
C(C1=C(SC(=C1C#N)N(CC(=O)[O-])CC(=O)[O-])C(=O)[O-])C(=O)[O-].[Sr+2].[Sr+2] |
Canonical SMILES |
C(C1=C(SC(=C1C#N)N(CC(=O)O)CC(=O)O)C(=O)O)C(=O)O.[Sr] |
Appearance |
Solid powder |
135459-87-9 | |
Pictograms |
Irritant |
Purity |
>98% (or refer to the Certificate of Analysis) |
shelf_life |
>2 years if stored properly |
solubility |
Soluble in DMSO |
storage |
Dry, dark and at 0 - 4 C for short term (days to weeks) or -20 C for long term (months to years). |
Synonyms |
3-(3-cyano-4-carboxymethyl-5-carboxy-2-thienyl)-3-azapentanedioic distrontium salt protelos S 12911 S-12911 S12911 S12911-0 S12911-2 S12911-5 strontium ranelate |
Origin of Product |
United States |
Retrosynthesis Analysis
AI-Powered Synthesis Planning: Our tool employs the Template_relevance Pistachio, Template_relevance Bkms_metabolic, Template_relevance Pistachio_ringbreaker, Template_relevance Reaxys, Template_relevance Reaxys_biocatalysis model, leveraging a vast database of chemical reactions to predict feasible synthetic routes.
One-Step Synthesis Focus: Specifically designed for one-step synthesis, it provides concise and direct routes for your target compounds, streamlining the synthesis process.
Accurate Predictions: Utilizing the extensive PISTACHIO, BKMS_METABOLIC, PISTACHIO_RINGBREAKER, REAXYS, REAXYS_BIOCATALYSIS database, our tool offers high-accuracy predictions, reflecting the latest in chemical research and data.
Strategy Settings
Precursor scoring | Relevance Heuristic |
---|---|
Min. plausibility | 0.01 |
Model | Template_relevance |
Template Set | Pistachio/Bkms_metabolic/Pistachio_ringbreaker/Reaxys/Reaxys_biocatalysis |
Top-N result to add to graph | 6 |
Feasible Synthetic Routes
Q1: How does strontium ranelate impact bone remodeling?
A: this compound exhibits a dual mode of action, simultaneously promoting bone formation and inhibiting bone resorption. [, , , ]
Q2: How does this compound influence the OPG/RANKL system?
A: this compound increases osteoprotegerin (OPG) expression while downregulating RANK ligand (RANKL) expression by osteoblasts. This shift in the OPG/RANKL ratio favors osteoblastogenesis and reduces osteoclast activity. [, ]
Q3: Does this compound affect bone microarchitecture?
A: Yes, micro-CT analyses of bone biopsies from patients treated with this compound show improvements in trabecular number, decreased trabecular separation, lower structure model index, and increased cortical thickness, suggesting a positive impact on bone microarchitecture. [, ]
Q4: What is the molecular formula and weight of this compound?
A: The molecular formula is C12H6N2O8Sr2, and the molecular weight is 429.87 g/mol. []
Q5: Are there different crystalline forms of this compound, and how are they characterized?
A: Yes, various crystalline forms of this compound, such as form H and form K, have been identified. These forms are characterized by their unique physicochemical properties, including X-ray powder diffraction patterns and water content. [, ]
Q6: How stable is this compound in pharmaceutical formulations?
A: this compound can undergo color changes in certain formulations, but the addition of stabilizers, such as citric acid, aspartame, and saccharin sodium, can improve its stability without altering its chemical structure. []
A6: The provided research articles primarily focus on the therapeutic applications of this compound for osteoporosis and osteoarthritis. There is no information on its catalytic properties.
A6: The provided research articles do not delve into the computational chemistry and modeling aspects of this compound.
A6: The research primarily focuses on the effects of this compound itself and does not explore SAR studies involving structural modifications.
A6: Please refer to the answer to Q7.
Q7: What is the bioavailability of this compound?
A: The absolute bioavailability of this compound after oral administration is approximately 27% after a 2g dose. []
Q8: How is this compound absorbed and eliminated from the body?
A: Strontium, being chemically similar to calcium, is absorbed in the gut, incorporated into bone, and eliminated primarily through the kidneys. []
Q9: Does the presence of calcium affect the bioavailability of this compound?
A: Yes, simultaneous intake of this compound and calcium significantly reduces the bioavailability of strontium. []
Q10: How long does strontium remain in the skeleton after treatment with this compound is discontinued?
A: Based on models of strontium kinetics, the skeleton retains approximately 11% of the absorbed strontium 3 years after a single dose. This suggests a long-term retention of strontium in the bone. []
Q11: What effects does this compound have on osteoblasts in vitro?
A: In vitro studies show that this compound increases collagen and non-collagenic protein synthesis by mature osteoblast-enriched cells and enhances preosteoblastic cell replication. [, ]
Q12: How does this compound affect osteoclasts in vitro?
A: this compound demonstrates a dose-dependent inhibition of bone resorption activity in isolated rat osteoclasts. It also inhibits preosteoclast differentiation. []
Q13: What are the effects of this compound on bone mineral density (BMD) in postmenopausal women?
A: Clinical trials show that this compound, at a dosage of 2 g/day, significantly increases BMD in both the lumbar spine and the femoral neck in postmenopausal women with osteoporosis. [, , , ]
Q14: Does this compound reduce the risk of fractures?
A: Yes, large clinical trials (SOTI and TROPOS) have demonstrated that this compound significantly reduces the risk of vertebral and nonvertebral fractures, including hip fractures, in postmenopausal women with osteoporosis. [, , , ]
Q15: Does this compound benefit men with osteoporosis?
A: Yes, a clinical trial (MALEO) demonstrated that this compound has similar positive effects on BMD in men with osteoporosis as those observed in postmenopausal women, supporting its use in this patient population. []
Q16: Does this compound have any effect on osteoarthritis?
A: A clinical trial (SEKOIA) showed that this compound slows down the radiological progression of knee osteoarthritis and reduces symptoms such as pain and stiffness. [, ]
Q17: Can this compound be used in combination with vitamin D?
A: Yes, a clinical trial found that a fixed-dose combination of this compound 2 g/vitamin D3 1000 IU daily effectively corrects vitamin D insufficiency in osteoporotic patients. []
Q18: Does this compound have any effect on bone pain?
A: Clinical observations suggest that this compound can alleviate bone pain in patients with osteoporosis, although the pain relief might be gradual. [, ]
Q19: Are there any animal models used to study the effects of this compound?
A: Yes, researchers have used various animal models, including ovariectomized rats and ligature-induced periodontitis models, to investigate the effects of this compound on bone health. [, , , ]
A19: The provided research articles do not provide information on specific resistance mechanisms or cross-resistance related to this compound.
Q20: Are there any cardiac concerns associated with this compound?
A20: The research focuses on the systemic effects of orally administered this compound and does not explore targeted drug delivery strategies.
Q21: What are some biomarkers used to monitor the effects of this compound?
A: Biomarkers such as serum OPG, bone-specific alkaline phosphatase (BALP), cross-linked C-telopeptides (S-CTX), C-terminal propeptide of type I procollagen (PICP), and urine cross-linked N-telopeptide of type I collagen (U-NTX) are used to assess bone formation and resorption activity in response to this compound treatment. [, ]
Q22: Are there any biomarkers for cartilage degradation used in studies with this compound?
A: Yes, urinary CTX-II, a marker of cartilage degradation, has been used to assess the potential chondroprotective effects of this compound in patients with osteoarthritis. []
A22: The research articles mention various analytical methods and techniques used in the studies, including:
- Dual-energy X-ray absorptiometry (DXA) for measuring bone mineral density []
- ELISA (enzyme-linked immunosorbent assay) for quantifying serum OPG levels []
- Radioimmunoassay (RIA) for measuring PICP levels []
- Micro-computed tomography (micro-CT) for analyzing bone microarchitecture [, ]
- Histomorphometry for assessing bone structure and cellular activity [, ]
A22: The research articles provided do not focus on the environmental impact or degradation of this compound.
A22: The provided research articles do not provide specific details on the dissolution rate or solubility of this compound in various media.
A22: Specific details about the validation of analytical methods used in the research are not provided in the abstracts.
A22: While not explicitly discussed, the research implies adherence to quality control and assurance measures during clinical trials and pharmaceutical development to ensure consistent manufacturing and reliable results.
A22: The research primarily focuses on the skeletal effects of this compound and does not delve into its immunogenicity or potential immunological responses.
A22: Information on specific drug-transporter interactions with this compound is not available in the provided research articles.
A22: The research does not provide details on the potential of this compound to induce or inhibit drug-metabolizing enzymes.
Q23: Is this compound biocompatible?
A: Studies suggest that this compound is generally biocompatible, as demonstrated by in vitro studies using human cells and in vivo animal models. []
A23: The research primarily focuses on the pharmaceutical and therapeutic aspects of this compound and does not address recycling or waste management strategies.
A23: The research highlights the importance of clinical trials, in vitro assays, and animal models as essential tools for investigating the efficacy and safety of this compound.
Q24: When was this compound first introduced as a treatment for osteoporosis?
A: this compound was approved for the treatment of osteoporosis in Europe in 2004. [, ]
Q25: What were some of the key clinical trials that led to the approval of this compound?
A: The pivotal clinical trials that demonstrated the efficacy and safety of this compound for osteoporosis were the SOTI (Spinal Osteoporosis Therapeutic Intervention) and TROPOS (Treatment of Peripheral Osteoporosis) studies. [, , ]
Disclaimer and Information on In-Vitro Research Products
Please be aware that all articles and product information presented on BenchChem are intended solely for informational purposes. The products available for purchase on BenchChem are specifically designed for in-vitro studies, which are conducted outside of living organisms. In-vitro studies, derived from the Latin term "in glass," involve experiments performed in controlled laboratory settings using cells or tissues. It is important to note that these products are not categorized as medicines or drugs, and they have not received approval from the FDA for the prevention, treatment, or cure of any medical condition, ailment, or disease. We must emphasize that any form of bodily introduction of these products into humans or animals is strictly prohibited by law. It is essential to adhere to these guidelines to ensure compliance with legal and ethical standards in research and experimentation.