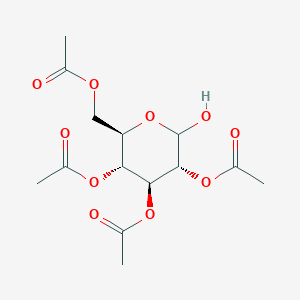
2,3,4,6-Tetra-O-acetyl-D-glucopyranose
- Click on QUICK INQUIRY to receive a quote from our team of experts.
- With the quality product at a COMPETITIVE price, you can focus more on your research.
Overview
Description
2,3,4,6-Tetra-O-acetyl-D-glucopyranose is an important raw material and intermediate used in organic synthesis, pharmaceuticals, agrochemicals, and dyestuff . It is a chemical compound with diverse therapeutic applications in diabetes, cancer, and HIV, and is a crucial building block in glycomics research .
Synthesis Analysis
The synthesis of 2,3,4,6-Tetra-O-acetyl-D-glucopyranose has been described in several studies . For instance, one protocol describes the synthesis of 2,3,4,6-tetra-O-acetyl-ß-d-glucopyranoside from 1,2,3,4,6-penta-O-acetyl-ß-d-glucopyranose and propargyl alcohol in the presence of boron trifluoride etherate .Molecular Structure Analysis
The molecular structure of 2,3,4,6-Tetra-O-acetyl-D-glucopyranose has been studied in several papers . The structure and reactivity of this compound unlock unparalleled opportunities for synthesizing complex carbohydrates .Chemical Reactions Analysis
The chemical reactions involving 2,3,4,6-Tetra-O-acetyl-D-glucopyranose have been studied . For example, one study discusses the inhibitory activity of compounds against some typical bacterial and fungal strains .Physical And Chemical Properties Analysis
2,3,4,6-Tetra-O-acetyl-D-glucopyranose is a white crystalline solid . It is soluble in methanol, ethanol, diethylether, acetone, dichloromethane, chloroform, tetrahydrofuran, benzene, and ethyl acetate .Scientific Research Applications
Preparation of Acyl-Gluco-, Galacto- and Mannopyranoses
2,3,4,6-Tetra-O-acetyl-D-glucopyranose is used in the preparation of acyl-gluco-, galacto- and mannopyranoses . The deacylation of glucose, galactose and mannose pentaacetates, as well as the dealkylation of 2,3,4,6-tetra-O-acetyl and 2,3,4,6-tetra-O-(3-bromo)benzoyl methyl α-D-glucopyranosides have been studied .
Synthesis of Thiosemicarbazones
It is used in the synthesis of thiosemicarbazones of 6-alkoxy-2-oxo-2H-chromene-4-carbaldehydes . These compounds exhibited remarkable antibacterial and antifungal activities against some typical bacteria and fungi .
Chiral Derivatization Reagent
2,3,4,6-Tetra-O-acetyl-β-D-glucopyranosyl isothiocyanate is a chiral derivatization reagent which reacts mainly with enantiomeric amino acids . This property makes it useful in the resolution of amino acid derivatives .
Preparation of Anionic Surfactants
Phosphorylated derivatives of 2,3,4,6-Tetra-O-acetyl-β-D-glucopyranose have proven valuable in the study of substrates for inositol synthase, and for the preparation of anionic surfactants .
Antibacterial and Antifungal Activities
The thiosemicarbazones synthesized using 2,3,4,6-Tetra-O-acetyl-D-glucopyranose showed remarkable antibacterial and antifungal activities against some typical bacteria and fungi .
Inhibition of DNA Gyrase
The thiosemicarbazones synthesized using 2,3,4,6-Tetra-O-acetyl-D-glucopyranose showed inhibitory activity against DNA gyrase, an enzyme that is essential for the replication of bacteria .
Future Directions
The future directions of research involving 2,3,4,6-Tetra-O-acetyl-D-glucopyranose are promising. It is a valuable intermediate towards the synthesis of heparin-like GAGs and is also a core intermediate relevant to the wider synthesis of other GlcN-containing targets . Its potential applications in diabetes, cancer, and HIV treatment are also being explored .
properties
IUPAC Name |
[(2R,3R,4S,5R)-3,4,5-triacetyloxy-6-hydroxyoxan-2-yl]methyl acetate |
Source
|
---|---|---|
Source | PubChem | |
URL | https://pubchem.ncbi.nlm.nih.gov | |
Description | Data deposited in or computed by PubChem | |
InChI |
InChI=1S/C14H20O10/c1-6(15)20-5-10-11(21-7(2)16)12(22-8(3)17)13(14(19)24-10)23-9(4)18/h10-14,19H,5H2,1-4H3/t10-,11-,12+,13-,14?/m1/s1 |
Source
|
Source | PubChem | |
URL | https://pubchem.ncbi.nlm.nih.gov | |
Description | Data deposited in or computed by PubChem | |
InChI Key |
IEOLRPPTIGNUNP-RQICVUQASA-N |
Source
|
Source | PubChem | |
URL | https://pubchem.ncbi.nlm.nih.gov | |
Description | Data deposited in or computed by PubChem | |
Canonical SMILES |
CC(=O)OCC1C(C(C(C(O1)O)OC(=O)C)OC(=O)C)OC(=O)C |
Source
|
Source | PubChem | |
URL | https://pubchem.ncbi.nlm.nih.gov | |
Description | Data deposited in or computed by PubChem | |
Isomeric SMILES |
CC(=O)OC[C@@H]1[C@H]([C@@H]([C@H](C(O1)O)OC(=O)C)OC(=O)C)OC(=O)C |
Source
|
Source | PubChem | |
URL | https://pubchem.ncbi.nlm.nih.gov | |
Description | Data deposited in or computed by PubChem | |
Molecular Formula |
C14H20O10 |
Source
|
Source | PubChem | |
URL | https://pubchem.ncbi.nlm.nih.gov | |
Description | Data deposited in or computed by PubChem | |
Molecular Weight |
348.30 g/mol |
Source
|
Source | PubChem | |
URL | https://pubchem.ncbi.nlm.nih.gov | |
Description | Data deposited in or computed by PubChem | |
Product Name |
2,3,4,6-Tetra-O-acetyl-D-glucopyranose |
Q & A
Q1: What is the significance of the anomeric center reactivity in 2,3,4,6-Tetra-O-acetyl-D-glucopyranoses?
A: Research on the iodination of monosaccharide derivatives [] shows that the anomeric center in 2,3,4,6-Tetra-O-acetyl-D-glucopyranoses exhibits varying reactivity depending on the substituent at the C-1 position. The rate of iodination decreases in the order: OAc > OBz ≈ OH >> OCH3. This selectivity is crucial for targeted modifications and synthesis of specific carbohydrate derivatives.
Q2: Can 2,3,4,6-Tetra-O-acetyl-D-glucopyranose be used to synthesize other important carbohydrate derivatives?
A: Yes, 2,3,4,6-Tetra-O-acetyl-D-glucopyranose serves as a versatile precursor for synthesizing various carbohydrate derivatives. For example, it can be converted to 2,3,4,6-tetra-O-acetyl-1-O-(methylthio)thiocarbonyl-D-glucopyranose through phase transfer methods []. This derivative is useful in carbohydrate chemistry for its ability to undergo further transformations.
Q3: Are there specific reaction conditions that favor the formation of particular derivatives from 2,3,4,6-Tetra-O-acetyl-D-glucopyranose?
A: Absolutely. The reaction conditions significantly influence the outcome of derivatization reactions. In the iodination study [], iodotrimethylsilane generated in situ was key for selective iodine introduction. Additionally, the choice of protecting groups on the sugar molecule, such as acetyl or benzoyl groups [], plays a vital role in directing the reaction pathway towards desired products.
Q4: Can 2,3,4,6-Tetra-O-acetyl-D-glucopyranose be used in the synthesis of naturally occurring compounds?
A: Yes, research shows that 2,3,4,6-Tetra-O-acetyl-D-glucopyranose can be employed in the synthesis of naturally occurring compounds like rothindin, an isoflavone glycoside []. The reaction utilizes 1-bromo-2,3,4,6-tetra-O-acetyl-D-glucopyranose and 7-hydroxy-3′,4′-methylenedioxyisoflavone (ψ-baptigenin) under specific conditions to produce the desired glycoside.
Disclaimer and Information on In-Vitro Research Products
Please be aware that all articles and product information presented on BenchChem are intended solely for informational purposes. The products available for purchase on BenchChem are specifically designed for in-vitro studies, which are conducted outside of living organisms. In-vitro studies, derived from the Latin term "in glass," involve experiments performed in controlled laboratory settings using cells or tissues. It is important to note that these products are not categorized as medicines or drugs, and they have not received approval from the FDA for the prevention, treatment, or cure of any medical condition, ailment, or disease. We must emphasize that any form of bodily introduction of these products into humans or animals is strictly prohibited by law. It is essential to adhere to these guidelines to ensure compliance with legal and ethical standards in research and experimentation.