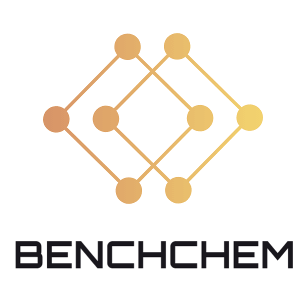
アロプリノール-d2
- 専門家チームからの見積もりを受け取るには、QUICK INQUIRYをクリックしてください。
- 品質商品を競争力のある価格で提供し、研究に集中できます。
概要
説明
Synthesis Analysis
The synthesis of Allopurinol involves various analytical methods available in the literature for the determination of allopurinol in pharmaceutical and biological samples consisting of HPLC, UV-visible method, near-IR spectroscopy, spectrofluorometry, capillary electrophoresis, polarography, voltammetry, and hyphenated techniques such as LC-MS, LC-MS/MS, UPLC-MS/MS, and GC-MS .Molecular Structure Analysis
Allopurinol is chemically 1H-pyrazolo [3,4-d]pyrimidin-4-ol. It is a tautomeric mixture of 1H-pyrazolo [3,4-d]pyrimidin-4-ol and 1,5-dihydro-4H-pyrazolo [3,4-d]pyrimidin-4-one . The molecular weight and molecular formula of Allopurinol are 136.11 g/mol and C5H4N4O, respectively .Chemical Reactions Analysis
Allopurinol can induce severe cutaneous adverse reactions (SCARs), including Drug Reaction with Eosinophilia and Systemic Symptoms (DRESS) syndrome, Stevens–Johnson syndrome (SJS) and toxic epidermal necrolysis (TEN) .Physical and Chemical Properties Analysis
Allopurinol is a white to almost white, crystalline powder . It is sparingly soluble in water and in ethanol (95%) and practically insoluble in chloroform and in ether . It is soluble in dilute alkali hydroxides solutions .科学的研究の応用
炎症性腸疾患(IBD)研究における役割
アロプリノールは、合成ヒポキサンチン異性体であり、炎症性腸疾患(IBD)に関連する実験的研究で使用されてきました。 アロプリノールはプリン代謝を阻害し、実験的結腸炎における損傷を増大させる可能性があります . アロプリノールは、キサンチンオキシダーゼによるヒポキサンチンの分解を阻害し、プリンサルベージも阻害します . これは、IBDの文脈における細胞代謝と腸の健康に大きな影響を与えます .
エネルギー代謝と創傷治癒への影響
研究によると、アロプリノールはエネルギー代謝と創傷治癒に影響を与える可能性があります。 ある研究では、治療的に関連するアロプリノールの投与量がアデニレートとクレアチンの代謝を変化させ、AMPKの調節不全と増殖の阻害につながることがわかりました . その結果、マウスの実験的結腸炎における創傷治癒の減弱と組織損傷の増加が見られました .
薬物動態/生物学的同等性研究における使用
アロプリノールとその活性代謝物であるオキシプリノールは、ヒト血漿中において、バリデーション済みの液体クロマトグラフィータンデム質量分析法を使用して同時に分析されています . この方法は、薬物動態/生物学的同等性研究で使用されています .
高尿酸血症および痛風治療における役割
アロプリノールは、高尿酸血症と痛風の治療に最も効果的で広く使用されている薬剤の1つです . その主な機能は、キサンチンオキシダーゼ酵素を阻害することです .
分析方法開発における使用
アロプリノールは、分析方法の開発に使用されてきました。 <a
作用機序
Target of Action
Allopurinol primarily targets the enzyme xanthine oxidase . This enzyme plays a crucial role in the conversion of hypoxanthine to xanthine and then to uric acid . By inhibiting xanthine oxidase, allopurinol prevents the formation of uric acid, which is the end product of purine metabolism .
Mode of Action
Allopurinol and its active metabolite, oxypurinol, inhibit the action of xanthine oxidase . They are also converted by the purine salvage pathway to their respective ribonucleotides . This interaction with the enzyme leads to a decrease in the production of uric acid without disrupting the biosynthesis of vital purines .
Biochemical Pathways
The inhibition of xanthine oxidase by allopurinol disrupts the biochemical pathway that leads to the formation of uric acid from hypoxanthine . This results in an increase in the levels of hypoxanthine and xanthine, which are more soluble and less likely to crystallize than uric acid . Furthermore, allopurinol can lead to the production of thioxanthine (2-hydroxymercaptopurine), which may inhibit thiopurine-S-methyltransferase (TPMT), providing a novel pathway of allopurinol-mediated TPMT inhibition .
Pharmacokinetics
Allopurinol has an oral bioavailability of approximately 79% and an elimination half-life of about 1.2 hours . It is rapidly and extensively metabolized to its active metabolite, oxypurinol . Oxypurinol has a longer half-life of around 23.3 hours and is cleared almost entirely by urinary excretion . The pharmacokinetics of allopurinol and oxypurinol are influenced by renal function, and dosage adjustments may be necessary in patients with renal impairment .
Result of Action
The primary result of allopurinol’s action is a reduction in the concentrations of uric acid in the blood and urine . This can help manage conditions like gout, where the deposition of uric acid crystals in body tissues leads to painful inflammation . By reducing uric acid production, allopurinol can decrease the frequency of gout attacks and prevent the formation of uric acid kidney stones .
Action Environment
The action of allopurinol can be influenced by various environmental factors. For instance, renal function significantly impacts the clearance of allopurinol and oxypurinol, affecting their concentrations and therapeutic effects . Additionally, the use of certain medications, such as uricosuric drugs, can affect the hypouricemic efficacy of allopurinol . These drugs increase the renal clearance of oxypurinol, potentially decreasing the influence of allopurinol . Therefore, the patient’s overall health status, concomitant medications, and other individual factors can influence the action, efficacy, and stability of allopurinol.
Safety and Hazards
将来の方向性
Allopurinol is often recommended as the first choice of treatment to control gout . If prescribed as soon as diagnosed, it may prevent future attacks and joint damage . More cohort studies are needed to determine an association between use of allopurinol and the probability of T2DM for individuals with gout and/or hyperuricemia .
生化学分析
Biochemical Properties
Allopurinol-d2, like its parent compound allopurinol, plays a significant role in biochemical reactions. It inhibits the enzyme xanthine oxidase, which is involved in the conversion of hypoxanthine to xanthine and xanthine to uric acid . This interaction with xanthine oxidase is non-competitive and results in a decrease in the production of uric acid .
Cellular Effects
Allopurinol-d2 impacts various types of cells and cellular processes. In human umbilical vein endothelial cells, allopurinol does not affect intracellular uric acid concentration . It also has an antioxidant property that might partially reverse endothelial dysfunction in patients with certain comorbidities .
Molecular Mechanism
The molecular mechanism of action of Allopurinol-d2 is primarily through its inhibition of the enzyme xanthine oxidase. This inhibition blocks the biochemical reactions that lead to the formation of uric acid . Allopurinol also has theoretical interest. In 1996, Begoña Hernández, Francisco J. Luque, and Modesto Orozco at the University of Barcelona used computational methods to evaluate the relative stabilities of tautomers of allopurinol and its isomer hypoxanthine .
Temporal Effects in Laboratory Settings
The effects of Allopurinol-d2 over time in laboratory settings have been observed in several studies. Allopurinol and its derivative have been shown to induce significant reduction in body weight, systolic blood pressure, blood glucose, insulin, lipids, and improved kidney functions and endothelial integrity compared to non-treated rats .
Dosage Effects in Animal Models
In animal models, the effects of Allopurinol-d2 vary with different dosages. For instance, in a study comparing the effects of febuxostat and allopurinol in a rat model of metabolic syndrome, it was found that febuxostat was more effective than allopurinol in normalizing serum fasting glucose, uric acid, catalase, and glutathione peroxidase activities .
Metabolic Pathways
Allopurinol-d2 is involved in purine metabolism, specifically in the catabolism of hypoxanthine and xanthine to uric acid. It inhibits the enzyme xanthine oxidase, thereby blocking this metabolic pathway . This leads to a decrease in the production of uric acid and an increase in the levels of hypoxanthine and xanthine .
Transport and Distribution
The transport and distribution of Allopurinol-d2 within cells and tissues are influenced by its interaction with various transporters. For example, in human umbilical vein endothelial cells, allopurinol does not affect intracellular uric acid concentration .
特性
{ "Design of the Synthesis Pathway": "The synthesis pathway for Allopurinol-d2 involves the conversion of commercially available starting materials to the final product through a series of chemical reactions.", "Starting Materials": [ "5-Chloro-1H-pyrazole", "Ethyl acetoacetate", "Hydrazine hydrate", "Sodium hydroxide", "2,6-Dichloropurine", "Deuterium oxide" ], "Reaction": [ "Step 1: React 5-Chloro-1H-pyrazole with Ethyl acetoacetate in the presence of Sodium hydroxide to obtain 5-Ethoxycarbonyl-4,6-dichloro-1H-pyrazole.", "Step 2: React 5-Ethoxycarbonyl-4,6-dichloro-1H-pyrazole with Hydrazine hydrate to obtain 5-Amino-4,6-dichloro-1H-pyrazole.", "Step 3: React 5-Amino-4,6-dichloro-1H-pyrazole with 2,6-Dichloropurine in the presence of Sodium hydroxide to obtain Allopurinol.", "Step 4: Deuterate Allopurinol using Deuterium oxide to obtain Allopurinol-d2." ] } | |
CAS番号 |
916979-34-5 |
分子式 |
C5H4N4O |
分子量 |
138.12 g/mol |
IUPAC名 |
3,6-dideuterio-1,2-dihydropyrazolo[3,4-d]pyrimidin-4-one |
InChI |
InChI=1S/C5H4N4O/c10-5-3-1-8-9-4(3)6-2-7-5/h1-2H,(H2,6,7,8,9,10)/i1D,2D |
InChIキー |
OFCNXPDARWKPPY-QDNHWIQGSA-N |
異性体SMILES |
[2H]C1=C2C(=NC(=NC2=O)[2H])NN1 |
SMILES |
C1=C2C(=NC=NC2=O)NN1 |
正規SMILES |
C1=C2C(=NC=NC2=O)NN1 |
同義語 |
1,5-Dihydro-4H-pyrazolo[3,4-d]pyrimidin-4-one-d2; 4-Hydroxypyrazolo[3,4-d]pyrimidine-d2; 4-Oxopyrazolo[3,4-d]pyrimidine-d2; Adenock-d2; Allopur-d2; Caplenal-d2; Cellidrin-d2; NSC 101655-d2; NSC 1390-d2; |
製品の起源 |
United States |
Retrosynthesis Analysis
AI-Powered Synthesis Planning: Our tool employs the Template_relevance Pistachio, Template_relevance Bkms_metabolic, Template_relevance Pistachio_ringbreaker, Template_relevance Reaxys, Template_relevance Reaxys_biocatalysis model, leveraging a vast database of chemical reactions to predict feasible synthetic routes.
One-Step Synthesis Focus: Specifically designed for one-step synthesis, it provides concise and direct routes for your target compounds, streamlining the synthesis process.
Accurate Predictions: Utilizing the extensive PISTACHIO, BKMS_METABOLIC, PISTACHIO_RINGBREAKER, REAXYS, REAXYS_BIOCATALYSIS database, our tool offers high-accuracy predictions, reflecting the latest in chemical research and data.
Strategy Settings
Precursor scoring | Relevance Heuristic |
---|---|
Min. plausibility | 0.01 |
Model | Template_relevance |
Template Set | Pistachio/Bkms_metabolic/Pistachio_ringbreaker/Reaxys/Reaxys_biocatalysis |
Top-N result to add to graph | 6 |
Feasible Synthetic Routes
試験管内研究製品の免責事項と情報
BenchChemで提示されるすべての記事および製品情報は、情報提供を目的としています。BenchChemで購入可能な製品は、生体外研究のために特別に設計されています。生体外研究は、ラテン語の "in glass" に由来し、生物体の外で行われる実験を指します。これらの製品は医薬品または薬として分類されておらず、FDAから任何の医療状態、病気、または疾患の予防、治療、または治癒のために承認されていません。これらの製品を人間または動物に体内に導入する形態は、法律により厳格に禁止されています。これらのガイドラインに従うことは、研究と実験において法的および倫理的な基準の遵守を確実にするために重要です。