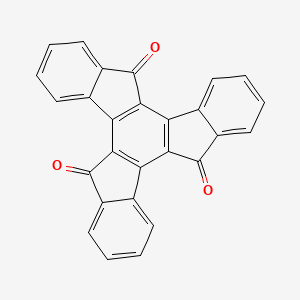
トルキセノン
概要
説明
科学的研究の応用
Truxenone has found extensive applications in scientific research, particularly in the following fields:
Biology: Research is ongoing to explore the potential biological activities of truxenone derivatives, including their use as probes for studying electron transfer processes in biological systems.
Medicine: While not extensively studied, truxenone derivatives hold potential as therapeutic agents due to their unique electronic properties.
作用機序
Target of Action
Truxenone is a versatile and widespread molecule that has been recognized in various fields such as liquid crystals and optoelectronics It’s known that truxenone and its derivatives have been used in organic photovoltaic applications as fullerene alternatives .
Mode of Action
Truxenone interacts with its targets primarily through its electron-accepting properties . These new electron-accepting molecules have easily tunable absorption profiles, more than ten-fold higher absorptivities than PCBM, slightly higher electron affinities than PCBM, and clearly defined and highly reversible reductive characteristics . The interaction of Truxenone with its targets results in changes in the electronic properties of the system, which can be leveraged for various applications .
Biochemical Pathways
Truxenone-based covalent organic frameworks (cofs) have been shown to boost photocatalytic hydrogen evolution . This suggests that Truxenone may influence pathways related to energy production and conversion.
Pharmacokinetics
It’s known that truxenone derivatives have promising optical properties with high molar extinction coefficients and easily tunable absorption maxima, while they also show good electrochemical stability during reduction with clearly defined electrochemical events .
Result of Action
The molecular and cellular effects of Truxenone’s action are primarily observed in its influence on optoelectronic properties and photocatalytic hydrogen evolution . For instance, Truxenone-based COFs have been shown to exhibit a high hydrogen evolution rate, resulting in a significant improvement in photocatalytic hydrogen evolution performance .
Action Environment
The action, efficacy, and stability of Truxenone can be influenced by environmental factors. For instance, the local charge delocalization and strong dipole moment are two key factors that affect the photocatalytic hydrogen evolution over Truxenone-based COFs . By controlling the structure and dipole of the monomers at the molecular level, the local charge delocalization can be evaluated, thereby significantly improving photocatalytic hydrogen evolution .
Safety and Hazards
将来の方向性
生化学分析
Biochemical Properties
Truxenone plays a significant role in biochemical reactions, particularly in the context of organic photovoltaics and photocatalysis. It interacts with various enzymes, proteins, and other biomolecules. For instance, truxenone derivatives have been shown to interact with electron-accepting molecules, enhancing their absorption profiles and electron affinities . These interactions are crucial for the compound’s function as an electron acceptor in organic solar cells. Additionally, truxenone-based porous organic polymers have demonstrated interactions with lung cancer A549 cells, reducing their multiplication through visible-light-induced photocatalysis .
Cellular Effects
Truxenone influences various cellular processes, including cell signaling pathways, gene expression, and cellular metabolism. In lung cancer A549 cells, truxenone-based polymers have been shown to reduce cell proliferation through photocatalytic activity . This effect is mediated by the generation of reactive intermediates under visible light, which subsequently impact cellular functions and gene expression.
Molecular Mechanism
The molecular mechanism of truxenone involves its interaction with biomolecules through electron-accepting properties. Truxenone derivatives exhibit highly reversible reductive characteristics, making them effective in electron transfer processes . In photocatalytic applications, truxenone-based polymers generate reactive intermediates that facilitate the functionalization of chemical feedstocks, impacting gene expression and enzyme activity in targeted cells .
Temporal Effects in Laboratory Settings
In laboratory settings, the effects of truxenone have been observed to change over time. The stability and degradation of truxenone-based polymers are crucial for their long-term efficacy. Studies have shown that these polymers can be reused multiple times without significant loss of activity, indicating their stability and sustained impact on cellular functions
Dosage Effects in Animal Models
The effects of truxenone vary with different dosages in animal models. At lower doses, truxenone-based compounds may exhibit beneficial effects, such as reducing cancer cell proliferation. At higher doses, there may be toxic or adverse effects, necessitating careful dosage optimization in experimental settings . Threshold effects and potential toxicity must be thoroughly evaluated to ensure safe and effective use.
Metabolic Pathways
Truxenone is involved in various metabolic pathways, interacting with enzymes and cofactors that facilitate its function. The compound’s electron-accepting properties play a crucial role in these pathways, impacting metabolic flux and metabolite levels
Transport and Distribution
Within cells and tissues, truxenone is transported and distributed through interactions with transporters and binding proteins. These interactions influence the compound’s localization and accumulation, affecting its overall efficacy . Understanding the transport mechanisms is essential for optimizing truxenone’s therapeutic and industrial applications.
Subcellular Localization
Truxenone’s subcellular localization is influenced by targeting signals and post-translational modifications. These factors direct the compound to specific compartments or organelles, impacting its activity and function
準備方法
Synthetic Routes and Reaction Conditions: Truxenone can be synthesized through several methods, one of which involves the Knoevenagel reaction. This reaction typically involves the condensation of 5-hexyl-2-thienyl-substituted truxenone with ethyl cyanoacetate under specific conditions to yield mono-, bis-, and tris-adducts . The yields of these adducts vary depending on the reaction conditions, with mono-adducts obtained in yields of 4-19%, bis-adducts in 28-31%, and tris-adducts in 14-23% .
Industrial Production Methods: Industrial production of truxenone often involves the epitaxial growth of truxenone monolayers on metallic surfaces such as copper (111). This method utilizes scanning tunneling microscopy and low energy electron diffraction to achieve commensurate epitaxial relationships between the molecular surface mesh and the substrate . This approach ensures the formation of highly ordered structures, which are crucial for optimal performance in electronic devices.
化学反応の分析
Types of Reactions: Truxenone undergoes various chemical reactions, including:
Reduction: The reduction of truxenone typically involves the use of reducing agents to convert the ketone groups into alcohols.
Common Reagents and Conditions:
Oxidation: Common oxidizing agents include potassium permanganate and chromium trioxide.
Reduction: Reducing agents such as lithium aluminum hydride and sodium borohydride are frequently used.
Substitution: Reagents like halogens and organometallic compounds are employed for substitution reactions.
Major Products: The major products formed from these reactions include various truxenone derivatives with modified electronic properties, which are tailored for specific applications in organic electronics and photovoltaics .
類似化合物との比較
- Phenyl-C61-butyric acid methyl ester (PCBM)
- Subphthalocyanine (SubPc)
- Other fullerene derivatives
特性
IUPAC Name |
heptacyclo[18.7.0.02,10.03,8.011,19.012,17.021,26]heptacosa-1(20),2(10),3,5,7,11(19),12,14,16,21,23,25-dodecaene-9,18,27-trione | |
---|---|---|
Source | PubChem | |
URL | https://pubchem.ncbi.nlm.nih.gov | |
Description | Data deposited in or computed by PubChem | |
InChI |
InChI=1S/C27H12O3/c28-25-16-10-4-1-7-13(16)19-22(25)20-15-9-3-6-12-18(15)27(30)24(20)21-14-8-2-5-11-17(14)26(29)23(19)21/h1-12H | |
Source | PubChem | |
URL | https://pubchem.ncbi.nlm.nih.gov | |
Description | Data deposited in or computed by PubChem | |
InChI Key |
HSMDIUMGCHZHNI-UHFFFAOYSA-N | |
Source | PubChem | |
URL | https://pubchem.ncbi.nlm.nih.gov | |
Description | Data deposited in or computed by PubChem | |
Canonical SMILES |
C1=CC=C2C(=C1)C3=C(C2=O)C4=C(C5=C3C(=O)C6=CC=CC=C65)C(=O)C7=CC=CC=C74 | |
Source | PubChem | |
URL | https://pubchem.ncbi.nlm.nih.gov | |
Description | Data deposited in or computed by PubChem | |
Molecular Formula |
C27H12O3 | |
Source | PubChem | |
URL | https://pubchem.ncbi.nlm.nih.gov | |
Description | Data deposited in or computed by PubChem | |
DSSTOX Substance ID |
DTXSID90327556 | |
Record name | NSC666122 | |
Source | EPA DSSTox | |
URL | https://comptox.epa.gov/dashboard/DTXSID90327556 | |
Description | DSSTox provides a high quality public chemistry resource for supporting improved predictive toxicology. | |
Molecular Weight |
384.4 g/mol | |
Source | PubChem | |
URL | https://pubchem.ncbi.nlm.nih.gov | |
Description | Data deposited in or computed by PubChem | |
CAS No. |
4430-15-3 | |
Record name | NSC666122 | |
Source | EPA DSSTox | |
URL | https://comptox.epa.gov/dashboard/DTXSID90327556 | |
Description | DSSTox provides a high quality public chemistry resource for supporting improved predictive toxicology. | |
Retrosynthesis Analysis
AI-Powered Synthesis Planning: Our tool employs the Template_relevance Pistachio, Template_relevance Bkms_metabolic, Template_relevance Pistachio_ringbreaker, Template_relevance Reaxys, Template_relevance Reaxys_biocatalysis model, leveraging a vast database of chemical reactions to predict feasible synthetic routes.
One-Step Synthesis Focus: Specifically designed for one-step synthesis, it provides concise and direct routes for your target compounds, streamlining the synthesis process.
Accurate Predictions: Utilizing the extensive PISTACHIO, BKMS_METABOLIC, PISTACHIO_RINGBREAKER, REAXYS, REAXYS_BIOCATALYSIS database, our tool offers high-accuracy predictions, reflecting the latest in chemical research and data.
Strategy Settings
Precursor scoring | Relevance Heuristic |
---|---|
Min. plausibility | 0.01 |
Model | Template_relevance |
Template Set | Pistachio/Bkms_metabolic/Pistachio_ringbreaker/Reaxys/Reaxys_biocatalysis |
Top-N result to add to graph | 6 |
Feasible Synthetic Routes
Q1: What is the molecular structure of truxenone?
A1: Truxenone, also known as diindeno[1,2-a;1′,2′-c]fluorene-5,10,15-trione, possesses a unique, planar, C3-symmetric structure with a central aromatic core composed of three indenone units fused together. []
Q2: What is the significance of truxenone's C3 symmetry?
A2: The C3 symmetry of truxenone is crucial for its self-assembly properties, enabling it to form ordered structures both in solution and on surfaces. [, , ] This makes truxenone a promising building block for supramolecular structures and organic electronic materials.
Q3: How do the electronic properties of truxenone compare to fullerene derivatives?
A3: Truxenone derivatives exhibit electron-deficient characteristics, making them suitable electron acceptors in organic photovoltaic devices. They possess similar electron affinities to fullerene derivatives like PCBM but boast significantly higher absorptivities and easily tunable absorption profiles. [, ]
Q4: What are the potential advantages of using truxenone derivatives over fullerenes in organic photovoltaics?
A4: Compared to fullerene-based acceptors, truxenone derivatives offer several advantages, including: * Enhanced light absorption: Truxenone derivatives display over ten times higher absorptivities than PCBM, enabling more efficient light harvesting in solar cells. [] * Tunable energy levels: The optoelectronic properties of truxenone derivatives can be easily fine-tuned by modifying the substituents on the core structure. [, ] * Defined redox behavior: These compounds exhibit well-defined and reversible reductive characteristics, crucial for efficient charge transfer processes in photovoltaic devices. []
Q5: What is the significance of the hydrogen bonding network observed in truxenone assemblies?
A5: The hydrogen bonding network formed between truxenone molecules on surfaces like highly oriented pyrolytic graphite (HOPG) contributes to the formation of large and near-perfect domains with excellent stability. [] This ordered arrangement is highly desirable for various applications, including molecular electronics and sensing.
Q6: How does truxenone self-assemble on surfaces?
A6: Truxenone exhibits diverse self-assembly behavior on surfaces, forming various two-dimensional supramolecular structures depending on the specific substrate and conditions. [] For instance, on Cu(111), truxenone forms both discrete islands and commensurate epitaxial monolayers. [, ]
Q7: Can the self-assembly of truxenone be controlled?
A7: Yes, the self-assembly of truxenone can be influenced by several factors, including:* Substrate: The choice of substrate directly impacts the packing motif of truxenone, leading to different two-dimensional structures. [, ]* Coverage: The density of truxenone molecules on the surface can affect the resulting packing arrangements, with distinct structures observed at low and high coverage. []* Presence of other molecules: Co-deposition with other molecules, such as copper phthalocyanine, can lead to the formation of binary superstructures with tunable molecular ratios. [, ]* Alkyl chain substitution: The pattern of alkyl chain substitution on the truxenone core significantly impacts both two-dimensional and three-dimensional self-assembly, affecting the packing arrangements and mesophase behavior. []
Q8: What is the role of truxenone in templated growth?
A8: Truxenone monolayers on suitable substrates, like Cu(111), can act as templates for the epitaxial growth of other molecules, such as C60 fullerene. [] This templating ability arises from the commensurate relationship between the molecular arrangement of truxenone and the substrate lattice.
Q9: How does truxenone contribute to the formation of chiral hierarchical structures?
A9: Truxenone, when combined with other molecules like copper phthalocyanine and trimesic acid homologues, can form intricate chiral hierarchical structures on surfaces. [, ] The specific interactions between the components and the underlying substrate drive the self-assembly process, leading to complex flower-like architectures with tunable pore sizes and chirality.
Q10: What are the potential applications of truxenone and its derivatives?
A10: Due to their unique properties, truxenone and its derivatives hold promise for diverse applications, including:* Organic photovoltaics: As efficient electron acceptors, truxenone derivatives can be utilized in organic solar cells to improve light harvesting and charge transport. [, , ]* Organic field-effect transistors (OFETs): The self-assembly properties of truxenone derivatives make them attractive for creating ordered thin films for use in OFETs. []* Sensors: Truxenone-based materials can serve as platforms for developing sensors for various analytes, with potential applications in environmental monitoring and biomedical diagnostics. [, ]* Nonlinear optics: The large first hyperpolarizabilities exhibited by some truxenone derivatives make them potential candidates for nonlinear optical applications. [, ]* Catalysis: Truxenone derivatives, particularly those incorporating metal centers, can be explored as potential catalysts for organic reactions. []
Q11: What are the challenges associated with using truxenone in practical applications?
A11: While promising, the use of truxenone in real-world applications faces challenges:* Synthesis and purification: Synthesizing specific truxenone derivatives with desired properties can be complex and require multi-step procedures. [, , ]* Stability: While truxenone itself is relatively stable, the stability of its derivatives can vary depending on the substituents. [] Further research is needed to enhance their long-term stability for device applications.* Processing: Developing efficient and scalable methods for processing truxenone-based materials into thin films or other desired forms is crucial for their practical implementation.
Q12: What insights have computational studies provided into the properties of truxenone?
A12: Computational methods like Density Functional Theory (DFT) have been instrumental in:* Understanding adsorption behavior: DFT calculations helped determine the preferred adsorption sites of truxenone on different surfaces, providing insights into its self-assembly mechanisms. [, ]* Explaining electronic properties: Calculations have elucidated the electronic structure of truxenone derivatives, rationalizing their observed electron-accepting capabilities and charge transport properties. [, ]* Predicting optical properties: Theoretical studies have aided in understanding the relationship between the structure of truxenone derivatives and their linear and nonlinear optical properties, guiding the design of new materials with tailored properties. [, , ]
Q13: How do structural modifications of truxenone influence its properties?
A13: Introducing various substituents onto the truxenone core can significantly alter its properties:* Electronic properties: Electron-donating or -withdrawing groups can tune the HOMO and LUMO energy levels, impacting its redox potential and charge transport characteristics. [, ]* Solubility: The addition of alkyl chains or other solubilizing groups can improve solubility in organic solvents, which is crucial for solution-processing techniques. [, ]* Self-assembly: Different substituents can influence the intermolecular interactions and packing arrangements of truxenone molecules, leading to distinct self-assembled structures. [, ]
Q14: Are there any QSAR models available for truxenone derivatives?
A14: While specific QSAR models for truxenone are limited, the structure-property relationships are actively being investigated. [] The growing body of experimental and computational data will facilitate the development of more accurate QSAR models, enabling the prediction of properties and rational design of new truxenone derivatives with optimized performance.
Q15: What is known about the environmental impact and degradation of truxenone?
A15: Currently, there is limited information available on the environmental fate and ecotoxicological effects of truxenone and its derivatives. Further research is necessary to assess their potential risks to the environment and develop appropriate waste management strategies. []
試験管内研究製品の免責事項と情報
BenchChemで提示されるすべての記事および製品情報は、情報提供を目的としています。BenchChemで購入可能な製品は、生体外研究のために特別に設計されています。生体外研究は、ラテン語の "in glass" に由来し、生物体の外で行われる実験を指します。これらの製品は医薬品または薬として分類されておらず、FDAから任何の医療状態、病気、または疾患の予防、治療、または治癒のために承認されていません。これらの製品を人間または動物に体内に導入する形態は、法律により厳格に禁止されています。これらのガイドラインに従うことは、研究と実験において法的および倫理的な基準の遵守を確実にするために重要です。