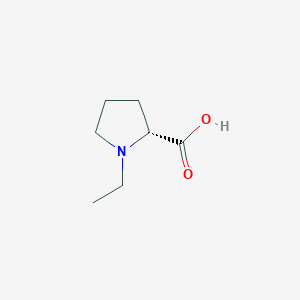
Ethyl-D-proline
概要
説明
Ethyl-D-proline is an isomer of the naturally occurring amino acid, L-Proline . D-amino acids have been found in relatively high abundance in human plasma and saliva . These amino acids may be of bacterial origin, but there is also evidence that they are endogenously produced through amino acid racemase activity .
Synthesis Analysis
L-Proline and D-Proline are often used as asymmetric organocatalysts for a variety of organic reactions . In a recent one-pot chiral synthesis, the Shi group used L/D-proline as a Lewis base, in the presence of a chiral auxiliary group to synthesize substituted isoxazoline-N-oxides .
Molecular Structure Analysis
The molecular weight of this compound is 143.19 . The IUPAC name is (2R)-1-ethyl-2-pyrrolidinecarboxylic acid .
Physical and Chemical Properties Analysis
This compound is a solid substance that should be stored at 2-8°C . It has a molecular weight of 143.19 .
科学的研究の応用
Catalysis and Synthesis
Ethyl-D-proline is involved in various catalytic and synthetic processes. For example, it's used in L-proline-catalyzed three-component domino reactions, contributing to the synthesis of highly substituted thieno[3,2-c]thiopyran derivatives. This process involves creating three C-C bonds and generating three new stereocenters in a single operation (Indumathi, Perumal, & Menéndez, 2010). Similarly, ethyl coumarin-3-carboxylate can be synthesized from aldehydes and diethyl malonate, using L-proline as a catalyst, a process that offers advantages like high yield and environmental friendliness (Lu Xiao-dong, 2012).
Biofuel Production
This compound plays a role in biofuel production. For instance, ethyl valerate, a potential fuel additive, can be produced from esterification of valeric acid with ethanol, catalyzed by amino acid ionic liquids like proline bisulfate (ProHSO4). This method achieves high conversion rates and shows potential for biofuel application (Dong, He, Tao, & Hu, 2013).
Polymer Science
In polymer science, studies have been conducted on the synthesis, characterization, and solution properties of 5‐ethyl‐substituted poly(L‐prolines), including cis‐ and trans‐5‐ethyl‐L‐proline. These studies aim to understand substituent effects on helical conformations and mutarotation in substituted poly(L-prolines) (Yang, Overberger, & Venkatachalam, 1983).
Agriculture and Plant Stress Resistance
This compound is implicated in plant stress resistance. For instance, ethephon, an ethylene-releasing compound, can mitigate nickel stress in plants by modulating antioxidant systems and enhancing proline metabolism, suggesting potential applications in agriculture (Khan et al., 2020).
Cryopreservation
In cryobiology, L-proline, including its derivatives, has been studied for its cryoprotective effects, particularly in mammalian oocyte cryopreservation. This research explores how L-proline can improve cryopreservation efficiency, potentially applicable to human oocyte vitrification (Zhang et al., 2016).
Medicinal Chemistry
In medicinal chemistry, L-proline and its derivatives, such as this compound, are utilized in the synthesis of various compounds. For example, L-proline can catalyze the synthesis of (E)-Ethyl 2-Cyano-3-(1H-indol-3-yl)acrylates, demonstrating its significance in creating biologically active molecules (Xiaoan Xie, Guorong Cai, & Dawei Ma, 2005).
作用機序
Target of Action
Ethyl-D-proline, an unnatural amino acid , is often used as an asymmetric organocatalyst for a variety of organic reactions . Its primary targets are likely to be the enzymes involved in these reactions. The specific targets may vary depending on the reaction context, but they generally involve enzymes that catalyze reactions where conformational rigidity is beneficial .
Mode of Action
This compound interacts with its targets by serving as a catalyst, facilitating the conversion of reactants to products in various organic reactions . Its conformational rigidity compared to other amino acids makes it particularly effective in this role . The resulting changes depend on the specific reaction, but they generally involve the synthesis of enantiopure compounds .
Biochemical Pathways
The biochemical pathways affected by this compound are those involving the organic reactions it catalyzes. For example, it has been used in asymmetric Mannich reactions for chiral β-aminocarbonyls, aldehyde aldol reactions for the synthesis of erythrose equivalents, and many others . The downstream effects of these reactions can be diverse, ranging from the synthesis of bioactive diketopiperazines to the formation of phosphodiester bond linkages to recognize DNA and RNAs .
Result of Action
The molecular and cellular effects of this compound’s action are largely dependent on the specific reactions it catalyzes. As a catalyst, it facilitates the conversion of reactants to products, potentially leading to the synthesis of new compounds with various biological activities . These compounds could then interact with cellular processes in a variety of ways.
Safety and Hazards
将来の方向性
The enantioselective aldol reaction is one of the most powerful methods for the construction of chiral polyol . The first intermolecular direct enantioselective aldol reaction catalyzed by L-proline appeared employing acetone and 4-nitrobenzaldehyde as the substrates . This result sparked high interest from several groups in further investigating proline-catalyzed direct asymmetric aldol reactions .
生化学分析
Biochemical Properties
Ethyl-D-proline plays a significant role in biochemical reactions, particularly as an asymmetric organocatalyst. It interacts with various enzymes and proteins, influencing their activity and stability. One notable interaction is with proline dehydrogenase, an enzyme involved in the proline degradation pathway. This compound can act as a substrate or inhibitor for this enzyme, affecting the overall proline metabolism . Additionally, this compound interacts with collagen, a structural protein, by incorporating into its structure and influencing its stability and function .
Cellular Effects
This compound has been shown to impact various cellular processes. It influences cell signaling pathways, particularly those involved in stress responses and apoptosis. For instance, this compound can modulate the activity of the mTOR pathway, which is crucial for cell growth and metabolism . Furthermore, this compound affects gene expression by altering the transcriptional activity of specific genes involved in cell cycle regulation and apoptosis . These changes can lead to alterations in cellular metabolism, including shifts in energy production and utilization.
Molecular Mechanism
At the molecular level, this compound exerts its effects through several mechanisms. It can bind to specific biomolecules, such as enzymes and receptors, altering their conformation and activity. For example, this compound has been shown to inhibit the activity of proline dehydrogenase by binding to its active site . This inhibition can lead to changes in proline metabolism and subsequent effects on cellular function. Additionally, this compound can influence gene expression by interacting with transcription factors and other regulatory proteins, leading to changes in the transcriptional landscape of the cell .
Temporal Effects in Laboratory Settings
In laboratory settings, the effects of this compound can change over time. The compound’s stability and degradation are critical factors influencing its long-term effects on cellular function. Studies have shown that this compound is relatively stable under physiological conditions, but it can degrade over time, leading to a decrease in its efficacy . Long-term exposure to this compound can result in sustained changes in cellular metabolism and function, including alterations in energy production and stress response pathways .
Dosage Effects in Animal Models
The effects of this compound vary with different dosages in animal models. At low doses, this compound can enhance cellular function and promote cell survival by modulating stress response pathways . At high doses, this compound can induce toxic effects, including oxidative stress and apoptosis . These adverse effects are likely due to the compound’s ability to disrupt cellular homeostasis and induce metabolic imbalances.
Metabolic Pathways
This compound is involved in several metabolic pathways, including the proline degradation pathway. It interacts with enzymes such as proline dehydrogenase and pyrroline-5-carboxylate reductase, influencing their activity and the overall flux of metabolites through these pathways . Additionally, this compound can affect the levels of key metabolites, such as proline and pyrroline-5-carboxylate, by modulating the activity of these enzymes .
Transport and Distribution
Within cells and tissues, this compound is transported and distributed through specific transporters and binding proteins. These transporters facilitate the uptake and localization of this compound within specific cellular compartments . The distribution of this compound can influence its activity and function, as it may accumulate in regions where its target enzymes and proteins are localized .
Subcellular Localization
This compound exhibits specific subcellular localization patterns, which can affect its activity and function. It is often found in the cytoplasm and mitochondria, where it interacts with enzymes involved in proline metabolism . The subcellular localization of this compound is influenced by targeting signals and post-translational modifications, which direct it to specific compartments within the cell . These localization patterns are crucial for its role in modulating cellular metabolism and function.
特性
{ "Design of the Synthesis Pathway": "The synthesis of Ethyl-D-proline can be achieved through a multi-step process involving the conversion of starting materials into intermediate compounds, which are then further modified to yield the final product.", "Starting Materials": [ "L-aspartic acid", "Ethyl bromide", "Sodium hydroxide", "Hydrochloric acid", "Sodium bicarbonate", "Sodium chloride", "Water" ], "Reaction": [ "Step 1: L-aspartic acid is reacted with ethyl bromide in the presence of sodium hydroxide to yield ethyl L-aspartate.", "Step 2: Ethyl L-aspartate is hydrolyzed with hydrochloric acid to yield L-aspartic acid and ethanol.", "Step 3: L-aspartic acid is then converted to L-proline through a series of reactions involving the formation of an imide intermediate.", "Step 4: L-proline is then converted to D-proline through a stereospecific reduction using sodium borohydride.", "Step 5: D-proline is esterified with ethanol in the presence of hydrochloric acid to yield Ethyl-D-proline.", "Step 6: The product is purified through recrystallization using a mixture of water and sodium bicarbonate, followed by filtration and drying." ] } | |
CAS番号 |
165552-34-1 |
分子式 |
C7H14ClNO2 |
分子量 |
179.64 g/mol |
IUPAC名 |
(2R)-1-ethylpyrrolidine-2-carboxylic acid;hydrochloride |
InChI |
InChI=1S/C7H13NO2.ClH/c1-2-8-5-3-4-6(8)7(9)10;/h6H,2-5H2,1H3,(H,9,10);1H/t6-;/m1./s1 |
InChIキー |
UBUGOZFEGIKNLN-FYZOBXCZSA-N |
異性体SMILES |
CCN1CCC[C@@H]1C(=O)O.Cl |
SMILES |
CCN1CCCC1C(=O)O |
正規SMILES |
CCN1CCCC1C(=O)O.Cl |
配列 |
P |
製品の起源 |
United States |
Retrosynthesis Analysis
AI-Powered Synthesis Planning: Our tool employs the Template_relevance Pistachio, Template_relevance Bkms_metabolic, Template_relevance Pistachio_ringbreaker, Template_relevance Reaxys, Template_relevance Reaxys_biocatalysis model, leveraging a vast database of chemical reactions to predict feasible synthetic routes.
One-Step Synthesis Focus: Specifically designed for one-step synthesis, it provides concise and direct routes for your target compounds, streamlining the synthesis process.
Accurate Predictions: Utilizing the extensive PISTACHIO, BKMS_METABOLIC, PISTACHIO_RINGBREAKER, REAXYS, REAXYS_BIOCATALYSIS database, our tool offers high-accuracy predictions, reflecting the latest in chemical research and data.
Strategy Settings
Precursor scoring | Relevance Heuristic |
---|---|
Min. plausibility | 0.01 |
Model | Template_relevance |
Template Set | Pistachio/Bkms_metabolic/Pistachio_ringbreaker/Reaxys/Reaxys_biocatalysis |
Top-N result to add to graph | 6 |
Feasible Synthetic Routes
Q1: What are the key structural features of poly(cis-5-ethyl-D-proline) and how do they influence its conformation?
A1: Poly(cis-5-ethyl-D-proline) exhibits two distinct helical conformations, similar to poly(L-proline), designated as form I and form II. These forms differ in the isomerism of the amide bond (cis for form I and trans for form II) [, ]. The presence of the ethyl group at the C5 position introduces steric effects that influence the preferred conformation. Specifically, the ethyl group prefers an anti conformation relative to the C5H bond, regardless of the overall form adopted by the polymer []. Additionally, in form II, the carbonyl group of the amide appears to be closer to a perpendicular orientation with respect to the helical axis compared to poly(L-proline) form II [].
Q2: Can the conformation of poly(cis-5-ethyl-D-proline) be altered?
A2: Yes, poly(cis-5-ethyl-D-proline) can undergo a process called mutarotation, transitioning between form I and form II. This process is influenced by hydrogen-bond-forming solvents, with the rate seemingly proportional to the solvent's acidity []. The carbonyl group of the amide has been identified as the specific site for hydrogen bonding, playing a crucial role in the mutarotation mechanism [].
Q3: What spectroscopic techniques are useful for studying the conformation of poly(cis-5-ethyl-D-proline)?
A3: Researchers have successfully employed a combination of spectroscopic techniques to elucidate the conformational characteristics of poly(cis-5-ethyl-D-proline). These techniques include:
- Nuclear Magnetic Resonance (NMR) Spectroscopy: Both ¹H-NMR and ¹³C-NMR provide valuable information about the local environment and interactions of atoms within the molecule, aiding in the identification and characterization of different conformations [].
- Circular Dichroism (CD) Spectroscopy: This technique helps determine the secondary structure of the polymer by analyzing the differential absorption of left- and right-circularly polarized light [].
- Optical Rotatory Dispersion (ORD) Spectroscopy: Similar to CD, ORD examines the variation of optical rotation with wavelength, providing insights into the molecule's chiral properties and conformation [].
- Infrared (IR) Spectroscopy: This technique aids in identifying specific functional groups and their interactions, such as the hydrogen bonding observed between the solvent and the carbonyl group of the amide during mutarotation [].
Q4: Are there theoretical approaches to study the conformational behavior of ethyl-substituted polyprolines?
A4: Yes, theoretical conformational energy calculations have been performed to understand the steric effects of substituents like ethyl groups on the preferred conformations of polyprolines []. These calculations can provide valuable insights into the energetic landscape of different conformations and guide experimental investigations.
試験管内研究製品の免責事項と情報
BenchChemで提示されるすべての記事および製品情報は、情報提供を目的としています。BenchChemで購入可能な製品は、生体外研究のために特別に設計されています。生体外研究は、ラテン語の "in glass" に由来し、生物体の外で行われる実験を指します。これらの製品は医薬品または薬として分類されておらず、FDAから任何の医療状態、病気、または疾患の予防、治療、または治癒のために承認されていません。これらの製品を人間または動物に体内に導入する形態は、法律により厳格に禁止されています。これらのガイドラインに従うことは、研究と実験において法的および倫理的な基準の遵守を確実にするために重要です。