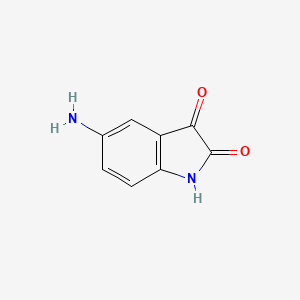
5-Aminoisatin
概要
説明
5-aminoisatin is isatin substituted at C-5 by an amino group. It derives from an isatin.
科学的研究の応用
Isatin Binding Proteins in Brain Research
Isatin, an endogenous indole, exhibits a range of pharmacological activities, and its specific biological targets are not well characterized. A study by Buneeva et al. (2010) utilized affinity chromatography and proteomic analysis to identify isatin-binding proteins in mouse and rat brains, using 5-aminoisatin as an affinity ligand. They discovered numerous proteins, and these findings could enhance our understanding of isatin's role in the brain and its pharmacological effects (Buneeva et al., 2010).
Protein Interaction Studies
Further investigation into isatin analogues' interactions with human proteins was conducted by Buneeva et al. (2010), who used an optical biosensor to study the binding of human recombinant cytokeratins with this compound. This study provides insights into the molecular interactions and affinities of isatin analogues with specific human proteins, aiding in understanding their biological significance (Buneeva et al., 2010).
Proteomic Profiling Using Affinity Sorbents
In 2012, Buneeva and colleagues conducted a study focusing on the proteomic profiling of isatin-binding proteins in rodent brains. They compared the profiles of proteins separated using this compound and another isatin analogue, revealing significant differences in the number and identity of proteins identified. This suggests that the choice of isatin analogue significantly influences the results of such proteomic studies, highlighting the importance of this compound in such research (Buneeva et al., 2012).
Synthesis and Evaluation of Isatin Derivatives
Ibrahim et al. (2021) explored the synthesis and evaluation of new isatin derivatives, starting from this compound, as potential tyrosine kinase inhibitors. This research contributes to the development of new pharmaceutical compounds with potential applications in cancer therapy (Ibrahim et al., 2021).
Computational Study on Isatin Derivatives
Czeleń, Szefler, and Skotnicka (2023) conducted a computational study on the immobilization of 5-nitroisatin derivatives using C60-based functionalized nanocarriers. This research is significant for developing new anti-cancer therapies, demonstrating the potential of this compound derivatives in targeted drug delivery and cancer treatment (Czeleń, Szefler, & Skotnicka, 2023).
Antitumor Effects of 5-Aminolevulinic Acid
Shishido et al. (2020) studied the antitumor effect of 5-aminolevulinic acid, a derivative of this compound, in esophageal squamous cell carcinoma. They found that this compound induces ferroptosis, a form of cell death, suggesting its potential as a new therapeutic agent for this type of cancer (Shishido et al., 2020).
Safety and Hazards
作用機序
Target of Action
5-Aminoisatin interacts with numerous isatin-binding proteins, which have been identified during proteomic profiling of mouse and rat brain preparations . Some of these proteins are involved in the development of neurodegenerative pathology .
Mode of Action
The mode of action of this compound is realized via interaction with these isatin-binding proteins .
Biochemical Pathways
It has been found that isatin, a related compound, can increase interaction between two human mitochondrial proteins, ferrochelatase (fech), and adrenodoxin reductase (adr), in a concentration-dependent manner .
Pharmacokinetics
It is known that isatin derivatives have been used experimentally for other diseases .
Result of Action
Administration of a neuroprotective dose of isatin, a related compound, led to a significant decrease in the total number of proteins bound to this compound . This suggests that this compound may have a similar effect.
生化学分析
Biochemical Properties
5-Aminoisatin plays a crucial role in several biochemical reactions. It interacts with enzymes such as monoamine oxidase and natriuretic peptide receptor guanylate cyclase. These interactions are pivotal in modulating the activity of these enzymes, thereby influencing various physiological processes. For instance, this compound inhibits monoamine oxidase, which is involved in the breakdown of neurotransmitters, thus affecting neurotransmission and mood regulation .
Cellular Effects
This compound exerts significant effects on various cell types and cellular processes. It influences cell signaling pathways, gene expression, and cellular metabolism. For example, this compound has been shown to induce apoptosis in certain cancer cell lines by modulating the expression of apoptosis-related genes. Additionally, it affects cell signaling pathways such as the natriuretic peptide signaling pathway, which plays a role in cardiovascular function .
Molecular Mechanism
At the molecular level, this compound exerts its effects through several mechanisms. It binds to specific biomolecules, leading to enzyme inhibition or activation. For instance, this compound inhibits monoamine oxidase by binding to its active site, thereby preventing the breakdown of neurotransmitters. This inhibition results in increased levels of neurotransmitters, which can influence mood and behavior. Additionally, this compound modulates gene expression by interacting with transcription factors and other regulatory proteins .
Temporal Effects in Laboratory Settings
In laboratory settings, the effects of this compound can vary over time. The compound is relatively stable, but its activity can diminish due to degradation. Long-term studies have shown that this compound can have sustained effects on cellular function, particularly in in vitro studies. For instance, prolonged exposure to this compound has been shown to induce apoptosis in cancer cells, suggesting potential therapeutic applications .
Dosage Effects in Animal Models
The effects of this compound vary with different dosages in animal models. At lower doses, this compound can modulate enzyme activity and influence metabolic pathways without causing significant toxicity. At higher doses, it can induce adverse effects such as hepatotoxicity and neurotoxicity. These threshold effects highlight the importance of careful dosage optimization in therapeutic applications .
Metabolic Pathways
This compound is involved in several metabolic pathways, including the monoamine oxidase pathway and the natriuretic peptide signaling pathway. It interacts with enzymes such as monoamine oxidase and natriuretic peptide receptor guanylate cyclase, influencing their activity and, consequently, the metabolic flux through these pathways. These interactions can lead to changes in metabolite levels and overall metabolic homeostasis .
Transport and Distribution
Within cells and tissues, this compound is transported and distributed through various mechanisms. It interacts with transporters and binding proteins that facilitate its movement across cell membranes and its accumulation in specific tissues. For instance, this compound has been shown to bind to plasma proteins, which can influence its distribution and bioavailability .
Subcellular Localization
The subcellular localization of this compound is crucial for its activity and function. It is directed to specific cellular compartments, such as the mitochondria and the nucleus, where it can exert its effects. Post-translational modifications and targeting signals play a role in directing this compound to these compartments. For example, this compound can be localized to the mitochondria, where it influences mitochondrial function and induces apoptosis in cancer cells .
特性
IUPAC Name |
5-amino-1H-indole-2,3-dione | |
---|---|---|
Source | PubChem | |
URL | https://pubchem.ncbi.nlm.nih.gov | |
Description | Data deposited in or computed by PubChem | |
InChI |
InChI=1S/C8H6N2O2/c9-4-1-2-6-5(3-4)7(11)8(12)10-6/h1-3H,9H2,(H,10,11,12) | |
Source | PubChem | |
URL | https://pubchem.ncbi.nlm.nih.gov | |
Description | Data deposited in or computed by PubChem | |
InChI Key |
NJTNUMGNMGRBNP-UHFFFAOYSA-N | |
Source | PubChem | |
URL | https://pubchem.ncbi.nlm.nih.gov | |
Description | Data deposited in or computed by PubChem | |
Canonical SMILES |
C1=CC2=C(C=C1N)C(=O)C(=O)N2 | |
Source | PubChem | |
URL | https://pubchem.ncbi.nlm.nih.gov | |
Description | Data deposited in or computed by PubChem | |
Molecular Formula |
C8H6N2O2 | |
Source | PubChem | |
URL | https://pubchem.ncbi.nlm.nih.gov | |
Description | Data deposited in or computed by PubChem | |
DSSTOX Substance ID |
DTXSID20473696 | |
Record name | 5-Aminoisatin | |
Source | EPA DSSTox | |
URL | https://comptox.epa.gov/dashboard/DTXSID20473696 | |
Description | DSSTox provides a high quality public chemistry resource for supporting improved predictive toxicology. | |
Molecular Weight |
162.15 g/mol | |
Source | PubChem | |
URL | https://pubchem.ncbi.nlm.nih.gov | |
Description | Data deposited in or computed by PubChem | |
CAS No. |
42816-53-5 | |
Record name | 5-Aminoisatin | |
Source | CAS Common Chemistry | |
URL | https://commonchemistry.cas.org/detail?cas_rn=42816-53-5 | |
Description | CAS Common Chemistry is an open community resource for accessing chemical information. Nearly 500,000 chemical substances from CAS REGISTRY cover areas of community interest, including common and frequently regulated chemicals, and those relevant to high school and undergraduate chemistry classes. This chemical information, curated by our expert scientists, is provided in alignment with our mission as a division of the American Chemical Society. | |
Explanation | The data from CAS Common Chemistry is provided under a CC-BY-NC 4.0 license, unless otherwise stated. | |
Record name | 5-Aminoisatin | |
Source | EPA DSSTox | |
URL | https://comptox.epa.gov/dashboard/DTXSID20473696 | |
Description | DSSTox provides a high quality public chemistry resource for supporting improved predictive toxicology. | |
Retrosynthesis Analysis
AI-Powered Synthesis Planning: Our tool employs the Template_relevance Pistachio, Template_relevance Bkms_metabolic, Template_relevance Pistachio_ringbreaker, Template_relevance Reaxys, Template_relevance Reaxys_biocatalysis model, leveraging a vast database of chemical reactions to predict feasible synthetic routes.
One-Step Synthesis Focus: Specifically designed for one-step synthesis, it provides concise and direct routes for your target compounds, streamlining the synthesis process.
Accurate Predictions: Utilizing the extensive PISTACHIO, BKMS_METABOLIC, PISTACHIO_RINGBREAKER, REAXYS, REAXYS_BIOCATALYSIS database, our tool offers high-accuracy predictions, reflecting the latest in chemical research and data.
Strategy Settings
Precursor scoring | Relevance Heuristic |
---|---|
Min. plausibility | 0.01 |
Model | Template_relevance |
Template Set | Pistachio/Bkms_metabolic/Pistachio_ringbreaker/Reaxys/Reaxys_biocatalysis |
Top-N result to add to graph | 6 |
Feasible Synthetic Routes
Q1: What are the primary targets of 5-Aminoisatin in the brain, and how does this interaction vary across species?
A1: Research has identified numerous proteins that bind to this compound in both mouse and rat brains. Interestingly, while the number of identified proteins was similar in both species, only about one-third were shared []. This suggests species-specific variations in this compound's targets and might contribute to the observed differences in its effects between rats and mice. One validated target, particularly within mitochondria, is the enzyme Monoamine Oxidase (MAO) [].
Q2: How do researchers study the interaction between this compound and its targets?
A2: Scientists utilize various techniques to study these interactions. Affinity chromatography, employing 5-aminocaproyl-isatin-Sepharose, helps isolate and identify proteins that bind to this compound []. Additionally, optical biosensors, such as the Biacore 3000, enable real-time monitoring of the interaction between this compound (or its analogues like 5-aminocaproyl-isatin) and its target proteins, providing valuable information about binding kinetics and affinity (Kd values) [, ].
Q3: What is the significance of this compound's interaction with Monoamine Oxidase (MAO)?
A3: MAO is an enzyme existing in two isoforms, MAO A and MAO B. Experiments using specific inhibitors, clorgyline for MAO A and deprenyl for MAO B, demonstrated a significant reduction in this compound binding to mitochondria []. This finding strongly suggests that MAO enzymes are major targets of this compound, particularly within the mitochondria.
Q4: How is this compound distributed within cells and tissues?
A4: this compound binding proteins are found in both membrane and soluble fractions of various tissues, including the brain, liver, heart, and kidneys []. Interestingly, the distribution pattern varies depending on the tissue and fraction analyzed. For instance, in the brain, heart, and liver, this compound binding proteins are predominantly located in the membrane fraction. Conversely, in the kidneys, these proteins are mainly found in the soluble fraction [].
Q5: Can the structure of this compound be modified to enhance its properties as a potential therapeutic agent?
A5: Yes, researchers are actively exploring structural modifications of this compound to improve its pharmacological profile []. This includes creating derivatives with enhanced binding affinity, selectivity for specific targets like tyrosine kinase, and improved pharmacokinetic properties. By combining this compound with other pharmacophores, scientists aim to develop multi-target drugs with superior therapeutic potential [].
Q6: What methods are used to synthesize and characterize new this compound derivatives?
A6: The synthesis of novel this compound derivatives often begins with commercially available this compound as the starting material [, ]. Various chemical reactions, such as amide formation and multicomponent reactions with carbonyl compounds like malononitrile and pyrazoline-2-one, are employed to introduce desired modifications to the this compound scaffold []. The synthesized compounds are then rigorously characterized using techniques like melting point determination, infrared spectroscopy (IR), and nuclear magnetic resonance spectroscopy (1H-NMR and 13C-NMR) to confirm their identity and purity [].
Q7: How do researchers predict the potential efficacy and safety of new this compound derivatives before moving to biological testing?
A7: Computational chemistry plays a crucial role in drug discovery by allowing researchers to predict the properties and behavior of molecules before conducting expensive and time-consuming experiments. Molecular docking studies, using software like GOLD Suite, help visualize and quantify the interaction between this compound derivatives and their target proteins, such as tyrosine kinase []. This provides insights into the binding affinity, binding mode, and potential selectivity of the compounds. Furthermore, in silico tools like SWISS ADME are employed to predict the pharmacokinetic profiles of the derivatives, including absorption, distribution, metabolism, and excretion (ADME) properties, and assess their drug-likeness based on Lipinski's rule of five []. This computational evaluation helps prioritize promising candidates with a higher likelihood of success in subsequent biological testing and minimizes potential risks associated with unfavorable pharmacological properties.
試験管内研究製品の免責事項と情報
BenchChemで提示されるすべての記事および製品情報は、情報提供を目的としています。BenchChemで購入可能な製品は、生体外研究のために特別に設計されています。生体外研究は、ラテン語の "in glass" に由来し、生物体の外で行われる実験を指します。これらの製品は医薬品または薬として分類されておらず、FDAから任何の医療状態、病気、または疾患の予防、治療、または治癒のために承認されていません。これらの製品を人間または動物に体内に導入する形態は、法律により厳格に禁止されています。これらのガイドラインに従うことは、研究と実験において法的および倫理的な基準の遵守を確実にするために重要です。