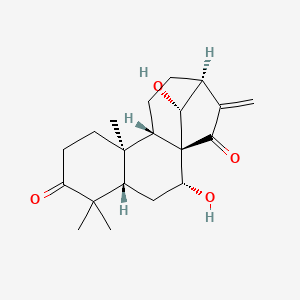
グラウコカリシンA
概要
説明
Synthesis Analysis
The total synthesis of GLA has been achieved through a series of complex organic reactions, highlighting its intricate molecular architecture. A notable synthesis approach involves Mn(OAc)3-mediated radical cyclization of alkynyl ketones, paving a new avenue for the synthesis of highly oxidized diterpenoids, including GLA. This method features a highly enantioselective conjugate addition/acylation cascade reaction, a Yamamoto aldol reaction, and an intramolecular Diels-Alder reaction to assemble the A/B ring system, demonstrating the synthetic challenge and complexity of GLA (Jia et al., 2020).
Molecular Structure Analysis
The molecular structure of GLA has been elucidated through comprehensive spectroscopic techniques, including IR, 1H NMR, 13C NMR, and X-ray crystallography. The structure is characterized by a tetracyclic system comprising three six-membered rings and a five-membered ring, with the six-membered rings in a chair-like conformation and the five-membered ring adopting a twisted envelope-like conformation. The molecular electrostatic potential map of GLA reveals key features necessary for its activity, suggesting sites for protonation on carbonyl oxygen atoms (Wang et al., 2009).
Chemical Reactions and Properties
GLA undergoes various chemical reactions, including oxidation and demethylation, leading to the formation of phase I and phase II metabolites. This bio-transformation is essential for its biological activity and includes conjugation with methylation, sulfate, and glucuronide to produce phase II metabolites. Such transformations are critical for understanding the pharmacological effects and mechanism of action of GLA (Sun et al., 2020).
Physical Properties Analysis
The physical properties of GLA, including its poor solubility in water, have posed significant challenges for its application. Strategies such as the formation of nanosuspensions have been explored to improve its solubility and bioavailability, enabling higher in vitro antitumor activity and indicating its potential for hepatic cancer treatment (Han et al., 2016).
Chemical Properties Analysis
The chemical properties of GLA, particularly its interaction with various signaling pathways, underscore its potential as a therapeutic agent. GLA has been shown to affect the PI3K/Akt pathway, NF-κB signaling, and the TGF-β1/Smad pathway, among others. These interactions contribute to its anti-inflammatory, anti-tumor, and cardioprotective effects, highlighting the diversity of its action mechanisms and its potential for further pharmacological exploration (Lin et al., 2018).
科学的研究の応用
急性腎障害 (AKI) の治療
GLA は、ヒト腎近位尿細管上皮細胞株 HK-2 細胞における低酸素/再酸素化誘発傷害を改善することが明らかになっています . これは、GLA が AKI の有望な治療薬となる可能性を示唆しています .
抗酸化作用
GLA は、抗酸化作用などの様々な薬理作用を持っています . HK-2 細胞における活性酸素種 (ROS) の産生を抑制することが明らかになっています .
免疫調節
GLA は、免疫調節にも役割を果たしています . HK-2 細胞におけるタンパク質キナーゼ B (Akt)/核内因子エリスロイド2関連因子 2 (Nrf2)/ヘムオキシゲナーゼ-1 (HO-1) シグナル伝達経路の活性化を改善することが明らかになっています .
抗アテローム性動脈硬化作用
GLA には抗アテローム性動脈硬化作用があります . ただし、この用途を完全に理解するためには、さらなる研究が必要です。
神経炎症の調節
GLA は、ミクログリアの活動を調節し、NF-kB および p38 MAPK シグナル伝達経路を介してリポ多糖誘発神経炎症を減弱させることが明らかになっています . これは、GLA がミクログリア媒介性神経炎症性疾患の治療のための潜在的な治療薬として開発できることを示唆しています .
アルツハイマー病の治療
GLA は、新しい mTOR 阻害剤として特定されており、アミロイド β の分解を増加させる可能性があり、アルツハイマー病の治療薬としての可能性を示唆しています .
高度に酸化されたジテルペノイドの合成
作用機序
Glaucocalyxin A (GLA) is an ent-kaurene diterpenoid extracted from the Chinese herb Rabdosia japonica var . It has been found to possess various beneficial biological activities, such as anti-cancer, anti-inflammatory, and immune regulation effects .
Target of Action
GLA primarily targets the HMGB3 protein and the STAT3 signaling pathway . HMGB3 is a protein that is often upregulated in epithelial ovarian cancer (EOC) tissues and cells . The STAT3 signaling pathway plays a crucial role in the progression of multiple myeloma .
Mode of Action
GLA interacts with its targets, leading to several changes in cellular processes. It dose-dependently inhibits EOC cell proliferation and epithelial-mesenchymal transition (EMT) . GLA also inhibits the phosphorylation of the signal transducer and activator of transcription 3 (STAT3) and downregulates interleukin IL-6 induced STAT3 phosphorylation in multiple myeloma .
Biochemical Pathways
GLA affects the miR-374b-5p/HMGB3/Wnt-β-Catenin pathway axis in EOC . Overexpression of miR-374b-5p, which is targeted by HMGB3, hinders malignant characteristics of EOC cells . Moreover, the Wnt-β-Catenin pathway is inhibited by the GLA-mediated miR-374b-5p/HMGB3 axis . In multiple myeloma, GLA blocks the activation of the STAT3 pathway .
Pharmacokinetics
GLA exhibits rapid absorption and elimination in rats after oral administration . The excretion of GLA is mainly as metabolites, with 23.8%, 12.8%, and 35.68% excreted in feces, urine, and bile within 12 hours, respectively .
Result of Action
GLA suppresses the malignant progression of EOC by regulating the miR-374b-5p/HMGB3/Wnt-β-Catenin pathway axis . It inhibits EOC tumor growth, upregulates the miR-374b-5p level, and downregulates the expression of HMGB3, Wnt3a, and β-catenin in tumor tissues . In multiple myeloma, GLA inhibits cell proliferation, induces cell apoptosis, and cell cycle arrest through blocking the activation of the STAT3 pathway .
Action Environment
The action of GLA can be influenced by environmental factors. For instance, glycosylation of GLA enhances its antitumor activity . This process modifies the conformation of the molecule, improving its solubility and orientation, and ultimately increasing its affinity and capacity as a biological receptor .
Safety and Hazards
将来の方向性
Glaucocalyxin A has shown potent anti-myeloma activity on MM cells . It has been suggested that Glaucocalyxin A may be a potential therapeutic candidate for multiple myeloma patients in the future . It has also been suggested as a promising natural medicine for non-small cell lung carcinoma therapy .
生化学分析
Biochemical Properties
Glaucocalyxin A plays a crucial role in various biochemical reactions. It interacts with several enzymes, proteins, and other biomolecules. For instance, Glaucocalyxin A has been shown to inhibit the activation of the NF-κB and MAPK signaling pathways, which are involved in inflammatory responses . Additionally, it interacts with proteins such as caspase-3, Bax, and Bcl-2, which are involved in the apoptotic pathway . These interactions highlight the compound’s potential in modulating inflammatory and apoptotic processes.
Cellular Effects
Glaucocalyxin A exerts significant effects on various types of cells and cellular processes. In human liver cancer cells, it has been shown to induce apoptosis and cell cycle arrest at the G2/M phase . The compound influences cell signaling pathways, such as the Akt and STAT3 pathways, leading to changes in gene expression and cellular metabolism . For example, Glaucocalyxin A treatment results in increased purine, pyrimidine, and sphingolipid metabolism, while decreasing amino acid metabolism . These effects demonstrate the compound’s potential in regulating cell proliferation and apoptosis.
Molecular Mechanism
The molecular mechanism of Glaucocalyxin A involves several key processes. It inhibits the phosphorylation of the signal transducer and activator of transcription 3 (STAT3) and downregulates interleukin IL-6 induced STAT3 phosphorylation . Additionally, Glaucocalyxin A induces apoptosis through the mitochondrial apoptotic pathway by increasing the Bax/Bcl-2 ratio, triggering reactive oxygen species (ROS) generation, reducing mitochondrial membrane potential, and inducing cleavage of caspase-9 and caspase-3 . These molecular interactions highlight the compound’s potential as an anticancer agent.
Temporal Effects in Laboratory Settings
In laboratory settings, the effects of Glaucocalyxin A change over time. The compound has been shown to be stable and effective in inhibiting cell proliferation and inducing apoptosis over extended periods Long-term studies have demonstrated that Glaucocalyxin A can maintain its protective effects on cartilage in osteoarthritis models for up to eight weeks . These findings suggest that the compound has sustained efficacy in laboratory settings.
Dosage Effects in Animal Models
The effects of Glaucocalyxin A vary with different dosages in animal models. In studies involving asthmatic mice, Glaucocalyxin A treatments reduced airway hyperresponsiveness and inflammation in a dose-dependent manner . High doses of the compound may lead to toxic or adverse effects, which necessitates careful dosage optimization in therapeutic applications. The compound’s protective effects on cartilage in osteoarthritis models were also observed at specific dosages .
Metabolic Pathways
Glaucocalyxin A is involved in various metabolic pathways. It undergoes oxidation and demethylation to produce phase I metabolites, and conjugation with methylation, sulfate, and glucuronide to produce phase II metabolites . These metabolic transformations highlight the compound’s extensive metabolism and potential interactions with metabolic enzymes. The compound’s effects on metabolic flux and metabolite levels in cancer cells have also been documented .
Transport and Distribution
Glaucocalyxin A is transported and distributed within cells and tissues through specific transporters and binding proteins. In glioblastoma cells, the compound was found to inhibit Akt phosphorylation and promote apoptosis
Subcellular Localization
The subcellular localization of Glaucocalyxin A plays a crucial role in its activity and function. The compound’s targeting signals and post-translational modifications direct it to specific compartments or organelles. For instance, Glaucocalyxin A has been shown to localize in the mitochondria, where it induces apoptosis through the mitochondrial apoptotic pathway . Understanding the subcellular localization of Glaucocalyxin A can provide insights into its mechanism of action and potential therapeutic applications.
特性
IUPAC Name |
(1R,2R,4S,9R,10S,13S,16R)-2,16-dihydroxy-5,5,9-trimethyl-14-methylidenetetracyclo[11.2.1.01,10.04,9]hexadecane-6,15-dione | |
---|---|---|
Source | PubChem | |
URL | https://pubchem.ncbi.nlm.nih.gov | |
Description | Data deposited in or computed by PubChem | |
InChI |
InChI=1S/C20H28O4/c1-10-11-5-6-12-19(4)8-7-14(21)18(2,3)13(19)9-15(22)20(12,16(10)23)17(11)24/h11-13,15,17,22,24H,1,5-9H2,2-4H3/t11-,12-,13+,15+,17+,19-,20-/m0/s1 | |
Source | PubChem | |
URL | https://pubchem.ncbi.nlm.nih.gov | |
Description | Data deposited in or computed by PubChem | |
InChI Key |
UCDVIBNDYLUWFP-MJTHGBBVSA-N | |
Source | PubChem | |
URL | https://pubchem.ncbi.nlm.nih.gov | |
Description | Data deposited in or computed by PubChem | |
Canonical SMILES |
CC1(C2CC(C34C(C2(CCC1=O)C)CCC(C3O)C(=C)C4=O)O)C | |
Source | PubChem | |
URL | https://pubchem.ncbi.nlm.nih.gov | |
Description | Data deposited in or computed by PubChem | |
Isomeric SMILES |
C[C@@]12CCC(=O)C([C@H]1C[C@H]([C@]34[C@H]2CC[C@H]([C@H]3O)C(=C)C4=O)O)(C)C | |
Source | PubChem | |
URL | https://pubchem.ncbi.nlm.nih.gov | |
Description | Data deposited in or computed by PubChem | |
Molecular Formula |
C20H28O4 | |
Source | PubChem | |
URL | https://pubchem.ncbi.nlm.nih.gov | |
Description | Data deposited in or computed by PubChem | |
Molecular Weight |
332.4 g/mol | |
Source | PubChem | |
URL | https://pubchem.ncbi.nlm.nih.gov | |
Description | Data deposited in or computed by PubChem | |
CAS RN |
79498-31-0 | |
Record name | Glaucocalyxin A | |
Source | CAS Common Chemistry | |
URL | https://commonchemistry.cas.org/detail?cas_rn=79498-31-0 | |
Description | CAS Common Chemistry is an open community resource for accessing chemical information. Nearly 500,000 chemical substances from CAS REGISTRY cover areas of community interest, including common and frequently regulated chemicals, and those relevant to high school and undergraduate chemistry classes. This chemical information, curated by our expert scientists, is provided in alignment with our mission as a division of the American Chemical Society. | |
Explanation | The data from CAS Common Chemistry is provided under a CC-BY-NC 4.0 license, unless otherwise stated. | |
Retrosynthesis Analysis
AI-Powered Synthesis Planning: Our tool employs the Template_relevance Pistachio, Template_relevance Bkms_metabolic, Template_relevance Pistachio_ringbreaker, Template_relevance Reaxys, Template_relevance Reaxys_biocatalysis model, leveraging a vast database of chemical reactions to predict feasible synthetic routes.
One-Step Synthesis Focus: Specifically designed for one-step synthesis, it provides concise and direct routes for your target compounds, streamlining the synthesis process.
Accurate Predictions: Utilizing the extensive PISTACHIO, BKMS_METABOLIC, PISTACHIO_RINGBREAKER, REAXYS, REAXYS_BIOCATALYSIS database, our tool offers high-accuracy predictions, reflecting the latest in chemical research and data.
Strategy Settings
Precursor scoring | Relevance Heuristic |
---|---|
Min. plausibility | 0.01 |
Model | Template_relevance |
Template Set | Pistachio/Bkms_metabolic/Pistachio_ringbreaker/Reaxys/Reaxys_biocatalysis |
Top-N result to add to graph | 6 |
Feasible Synthetic Routes
Q & A
Q1: What are the primary molecular targets of GLA?
A1: GLA exhibits its effects through interactions with various molecular targets. One prominent target is Akt, a serine/threonine-specific protein kinase implicated in cell survival, proliferation, and metabolism. GLA functions as a potent negative regulator of Akt, inhibiting its phosphorylation and downstream signaling. [] Additionally, GLA has been shown to influence other signaling pathways, including STAT3, NF-κB, TGF-β1/Smad, and ATF4/CHOP/CHAC1. [, , , ]
Q2: How does GLA's interaction with Akt influence cancer cell behavior?
A2: GLA's ability to inhibit Akt phosphorylation leads to a cascade of downstream effects that contribute to its antitumor activity. Specifically, GLA treatment has been shown to:
- Suppress Proliferation: By inhibiting Akt, GLA disrupts crucial signaling pathways involved in cell cycle progression, ultimately leading to reduced proliferation of various cancer cell lines, including glioblastoma, hepatocellular carcinoma, and oral squamous cell carcinoma. [, , ]
- Promote Apoptosis: GLA induces apoptosis, a programmed cell death mechanism, in cancer cells. This effect is attributed to the activation of caspase-3, decreased phosphorylation of BAD (Bcl-2-associated death promoter), and reduced expression of XIAP (X-linked inhibitor of apoptosis protein). []
- Inhibit EMT: Epithelial-mesenchymal transition (EMT) is a process that contributes to cancer cell invasion and metastasis. GLA has demonstrated the ability to suppress EMT by regulating the miR-374b-5p/HMGB3 axis, impacting the expression of EMT markers such as E-cadherin and N-cadherin. []
Q3: Beyond cancer, what other therapeutic effects are linked to GLA's modulation of signaling pathways?
A3: GLA's influence extends beyond antitumor activity to encompass:
- Anti-inflammatory Effects: GLA attenuates inflammatory responses in various models, including lipopolysaccharide-induced inflammation in pulmonary microvascular endothelial cells and interleukin-1β-induced inflammation in osteoarthritis chondrocytes. These effects are attributed to the suppression of pro-inflammatory cytokine production (e.g., IL-6, TNF-α) and the inhibition of NF-κB signaling. [, ]
- Antifibrotic Activity: Studies have shown that GLA can alleviate liver fibrosis by inhibiting the activation and proliferation of hepatic stellate cells (HSCs). This effect is linked to the suppression of TGF-β1/Smad signaling, a key pathway involved in HSC activation and extracellular matrix deposition. []
- Cardioprotective Effects: GLA exhibits protective effects against hypoxia/reoxygenation-induced injury in cardiomyocytes. This protection is attributed to the activation of the Akt/Nrf2/HO-1 pathway, leading to increased antioxidant enzyme activity and reduced oxidative stress. []
Q4: What is the molecular formula, weight, and key spectroscopic data for GLA?
A4: GLA is characterized by the following:
- IR Spectroscopy: Characteristic peaks corresponding to hydroxyl (OH) stretching, carbonyl (C=O) stretching, and alkene (C=C) stretching vibrations. []
- NMR Spectroscopy: Detailed 1H and 13C NMR data have been reported, providing insights into the structure and conformation of GLA. [, ]
Q5: What is known about the stability of GLA and strategies to improve its pharmaceutical properties?
A5: GLA exhibits limited water solubility, hindering its wider pharmaceutical applications. [] To address this, researchers have explored various formulation strategies:
- Solid Lipid Nanoparticles (SLNs): Encapsulating GLA within SLNs has demonstrated enhanced drug loading, improved stability, and sustained release profiles. This approach shows promise for enhancing GLA's bioavailability and therapeutic efficacy. [, ]
- Cyclodextrin Clathrates: Complexation of GLA with γ-cyclodextrin results in the formation of inclusion complexes (clathrates) that significantly enhance its solubility and dissolution rate, potentially improving its bioavailability. []
- Nanosuspensions: Preparing GLA nanosuspensions through precipitation combined with ultrasonication yielded particles with a small size and improved drug loading, leading to enhanced in vitro and in vivo antitumor activity compared to free GLA. []
Q6: What are the key pharmacokinetic parameters of GLA following different routes of administration?
A6: The pharmacokinetic profile of GLA has been investigated in rats, revealing:
- Intravenous Administration: Following a single intravenous dose (15 mg/kg), GLA exhibits a two-compartment open model with a short half-life (t1/2) and rapid clearance. []
Q7: What is the relationship between GLA's pharmacokinetic properties and its observed pharmacological effects?
A7: The relatively short half-life of GLA following intravenous administration suggests a need for frequent dosing or sustained-release formulations to maintain therapeutic levels. [] The improved oral absorption observed with Rabdosia japonica extract highlights the potential of synergistic interactions with other plant constituents. [] Further research is needed to fully elucidate the PK/PD relationship and optimize dosing regimens for different therapeutic applications.
Q8: What in vitro models have been used to evaluate the biological activity of GLA?
A8: A range of in vitro cell-based assays have been employed to investigate GLA's effects, including:
- Cytotoxicity Assays: MTT assays are commonly used to assess the cytotoxic effects of GLA on various cancer cell lines, revealing potent antiproliferative activity. [, , ]
- Apoptosis Assays: Flow cytometry analysis using Annexin V/PI staining, along with the evaluation of apoptotic markers (e.g., caspase-3, Bax, Bcl-2), has been used to demonstrate GLA's ability to induce apoptosis in cancer cells. [, , ]
- Cell Cycle Analysis: Flow cytometry analysis of cell cycle distribution reveals that GLA can induce cell cycle arrest, particularly in the G0/G1 phase, leading to inhibition of cell proliferation. []
- Migration and Invasion Assays: Transwell assays and wound healing assays have been used to demonstrate the ability of GLA to inhibit the migration and invasion of cancer cells, suggesting its potential to prevent metastasis. [, ]
- Inflammatory Response Assays: The production of inflammatory cytokines (e.g., IL-6, TNF-α) and the expression of inflammatory enzymes (e.g., iNOS, COX-2) have been measured in LPS-stimulated cells to evaluate the anti-inflammatory effects of GLA. [, , ]
Q9: What in vivo models have been used to validate the therapeutic potential of GLA?
A9: Several animal models have been employed to confirm the in vivo efficacy of GLA:
- Tumor Xenograft Models: Studies using subcutaneous xenograft models in mice have demonstrated that GLA significantly inhibits tumor growth, supporting its antitumor potential. [, , , ]
- Liver Fibrosis Models: In mice with carbon tetrachloride-induced liver fibrosis, GLA administration reduced liver injury markers, attenuated collagen deposition, and improved liver function, suggesting its potential as an antifibrotic agent. []
- Thrombosis Models: GLA has shown efficacy in reducing thrombus formation in rat models of arterial thrombosis, indicating its potential as an antithrombotic agent. []
Q10: What analytical methods have been employed to characterize, quantify, and monitor GLA?
A10: Various analytical techniques have been used to study GLA:
- High-Performance Liquid Chromatography (HPLC): HPLC, coupled with UV detection or mass spectrometry (MS), has been widely used for the quantitative determination of GLA in various matrices, including plant extracts, plasma, bile, urine, and feces. [, , , , ]
- Ultra-High-Performance Liquid Chromatography (UHPLC): UHPLC coupled with MS/MS offers enhanced sensitivity and resolution for the analysis of GLA and its metabolites in biological samples. [, ]
- Mass Spectrometry (MS): MS techniques, particularly in tandem with liquid chromatography (LC-MS/MS or UHPLC-MS/MS), have been instrumental in identifying and characterizing GLA metabolites in vitro and in vivo. [, ]
試験管内研究製品の免責事項と情報
BenchChemで提示されるすべての記事および製品情報は、情報提供を目的としています。BenchChemで購入可能な製品は、生体外研究のために特別に設計されています。生体外研究は、ラテン語の "in glass" に由来し、生物体の外で行われる実験を指します。これらの製品は医薬品または薬として分類されておらず、FDAから任何の医療状態、病気、または疾患の予防、治療、または治癒のために承認されていません。これらの製品を人間または動物に体内に導入する形態は、法律により厳格に禁止されています。これらのガイドラインに従うことは、研究と実験において法的および倫理的な基準の遵守を確実にするために重要です。