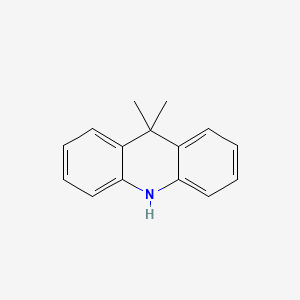
9,9-ジメチル-9,10-ジヒドロアクリジン
概要
説明
9,9-Dimethyl-9,10-dihydroacridine (DMDA) is a synthetic organic compound derived from acridine, a polycyclic aromatic compound. DMDA is a highly versatile compound with a wide range of applications in the fields of chemistry, biology, and medicine. DMDA is a colorless solid that is soluble in many organic solvents and has a melting point of 60-62°C. It has been used in a variety of research studies due to its unique properties, such as its low toxicity, low reactivity, and high solubility.
科学的研究の応用
有機発光ダイオード(OLED)
9,9-ジメチル-9,10-ジヒドロアクリジン: は、熱活性化遅延蛍光(TADF)を示す能力があるため、OLEDの開発に利用されてきました {svg_1}。この特性は、一重項励起子と三重項励起子の両方を収穫できるようにするため、OLEDの効率を高めるために不可欠です。 この化合物の誘導体は、534〜609 nmの範囲で高い熱安定性と発光を示し、幅広い色のデバイスを作成するために有利です {svg_2}。
光物理的メカニズム研究
この化合物の誘導体は合成され、その電子、光物理、電気化学的特性について研究されています。 これらの研究は、これらの化合物に見られる分子内電荷移動と正の溶媒変色性を理解するために不可欠であり、これらは高度な光物理的メカニズムを設計する際の重要な要因です {svg_3}。
高性能TADF材料
ポリマーの文脈では、9,9-ジメチル-9,10-ジヒドロアクリジンは、空間的電荷移動(TSCT)TADFを示すコポリマーのドナーモノマーとして組み込まれてきました。 これらの材料は、高度なディスプレイ技術など、高性能TADFを必要とする用途に有望です {svg_4}。
非共役ポリマー
この化合物は、開環重合によって非共役ポリマーの合成に使用されてきました。 これらのポリマーは、分子量が最大68.0 kDaであり、センサーやバイオイメージングなど、青緑色のTSCT TADFが有利な用途での可能性を示しています {svg_5}。
発光状態の分子設計
9,9-ジメチル-9,10-ジヒドロアクリジン: は、瞬時蛍光とTADFの利点を単一のエミッターに組み合わせることを目的とした分子設計の一部です。 このアプローチは、効率的で安定した発光材料を作成するために重要です {svg_6}。
凝集誘起発光(AIE)
この化合物は、凝集誘起発光(AIE)特性についても調査されています。 AIE活性材料は、その多様な用途、特にOLEDの分野で求められており、そこでデバイスの効率と安定性を向上させるのに貢献できます {svg_7}。
作用機序
Target of Action
The primary target of 9,9-Dimethyl-9,10-dihydroacridine is DNA . The compound interacts with DNA through a process known as intercalation . This process involves the insertion of the planar aromatic rings of the compound between the base pairs of the DNA double helix .
Mode of Action
The mode of action of 9,9-Dimethyl-9,10-dihydroacridine primarily involves DNA intercalation . The compound’s planar structure allows it to insert itself between the base pairs of the DNA double helix . This intercalation can disrupt the normal functioning of DNA and related enzymes, leading to various downstream effects .
Biochemical Pathways
The biochemical pathways affected by 9,9-Dimethyl-9,10-dihydroacridine are primarily those involving DNA and related enzymes . By intercalating into DNA, the compound can disrupt the normal functioning of these enzymes, potentially leading to a variety of downstream effects .
Result of Action
The result of the action of 9,9-Dimethyl-9,10-dihydroacridine is primarily the disruption of normal DNA function . By intercalating into DNA, the compound can disrupt the normal functioning of DNA and related enzymes . This can lead to a variety of downstream effects, potentially including the inhibition of cell growth and division .
Action Environment
The action of 9,9-Dimethyl-9,10-dihydroacridine can be influenced by various environmental factors. For example, the compound’s luminescent properties can be affected by the presence of other molecules, such as those found in organic light-emitting diodes (OLEDs) . Additionally, the compound’s stability and efficacy may be influenced by factors such as temperature and light exposure .
Safety and Hazards
生化学分析
Biochemical Properties
9,9-Dimethyl-9,10-dihydroacridine plays a significant role in biochemical reactions, particularly as a catalyst and a stabilizing agent. It interacts with various enzymes, proteins, and other biomolecules. For instance, it has been observed to interact with FtsZ, a protein involved in bacterial cell division, promoting FtsZ polymerization and disrupting the formation of the Z-ring at the division site . This interaction leads to the interruption of bacterial cell division and ultimately cell death. Additionally, 9,9-Dimethyl-9,10-dihydroacridine exhibits strong basicity, making it an effective organic base catalyst in various biochemical reactions .
Cellular Effects
The effects of 9,9-Dimethyl-9,10-dihydroacridine on various types of cells and cellular processes are profound. It influences cell function by affecting cell signaling pathways, gene expression, and cellular metabolism. For example, in bacterial cells, 9,9-Dimethyl-9,10-dihydroacridine disrupts cell division by promoting FtsZ polymerization, leading to cell death . In eukaryotic cells, it has been shown to enhance fluorescence properties, making it useful in super-resolution imaging of live cells . This compound also affects cellular metabolism by acting as a catalyst in various biochemical reactions, thereby influencing metabolic flux and metabolite levels .
Molecular Mechanism
At the molecular level, 9,9-Dimethyl-9,10-dihydroacridine exerts its effects through several mechanisms. It binds to specific biomolecules, such as FtsZ, promoting polymerization and disrupting cell division . Additionally, it acts as an enzyme inhibitor or activator, depending on the specific biochemical reaction. For instance, it inhibits the formation of reactive oxygen species (ROS) by preventing the oxidation of certain substrates . This inhibition is crucial in reducing oxidative stress and protecting cells from damage. Furthermore, 9,9-Dimethyl-9,10-dihydroacridine can modulate gene expression by interacting with transcription factors and other regulatory proteins .
Temporal Effects in Laboratory Settings
The stability and degradation of 9,9-Dimethyl-9,10-dihydroacridine over time have been studied extensively in laboratory settings. It has been found to be highly stable at room temperature, with minimal degradation or oxidation . Long-term studies have shown that 9,9-Dimethyl-9,10-dihydroacridine maintains its biochemical properties and continues to exert its effects on cellular function over extended periods . In in vitro and in vivo studies, the compound has demonstrated consistent activity, making it a reliable agent for various biochemical applications.
Dosage Effects in Animal Models
The effects of 9,9-Dimethyl-9,10-dihydroacridine vary with different dosages in animal models. At low doses, it has been observed to enhance cellular function and promote metabolic activity . At high doses, it can exhibit toxic or adverse effects, such as disrupting cellular homeostasis and causing oxidative stress . Threshold effects have been identified, indicating the optimal dosage range for achieving desired biochemical outcomes without causing harm to the organism.
Metabolic Pathways
9,9-Dimethyl-9,10-dihydroacridine is involved in several metabolic pathways, interacting with various enzymes and cofactors. It acts as a catalyst in the synthesis of amides and esters, as well as in acid-catalyzed reactions . The compound also influences metabolic flux by modulating the activity of key enzymes involved in metabolic pathways . These interactions can lead to changes in metabolite levels and overall metabolic activity.
Transport and Distribution
Within cells and tissues, 9,9-Dimethyl-9,10-dihydroacridine is transported and distributed through specific transporters and binding proteins. It has been shown to interact with membrane transporters, facilitating its uptake and distribution within the cell . The compound’s localization and accumulation are influenced by its interactions with these transporters and binding proteins, affecting its overall activity and function.
Subcellular Localization
The subcellular localization of 9,9-Dimethyl-9,10-dihydroacridine plays a crucial role in its activity and function. It has been observed to localize in specific cellular compartments, such as the cytoplasm and nucleus . This localization is directed by targeting signals and post-translational modifications that guide the compound to specific organelles. The subcellular distribution of 9,9-Dimethyl-9,10-dihydroacridine influences its interactions with biomolecules and its overall biochemical effects.
特性
IUPAC Name |
9,9-dimethyl-10H-acridine | |
---|---|---|
Source | PubChem | |
URL | https://pubchem.ncbi.nlm.nih.gov | |
Description | Data deposited in or computed by PubChem | |
InChI |
InChI=1S/C15H15N/c1-15(2)11-7-3-5-9-13(11)16-14-10-6-4-8-12(14)15/h3-10,16H,1-2H3 | |
Source | PubChem | |
URL | https://pubchem.ncbi.nlm.nih.gov | |
Description | Data deposited in or computed by PubChem | |
InChI Key |
JSEQNGYLWKBMJI-UHFFFAOYSA-N | |
Source | PubChem | |
URL | https://pubchem.ncbi.nlm.nih.gov | |
Description | Data deposited in or computed by PubChem | |
Canonical SMILES |
CC1(C2=CC=CC=C2NC3=CC=CC=C31)C | |
Source | PubChem | |
URL | https://pubchem.ncbi.nlm.nih.gov | |
Description | Data deposited in or computed by PubChem | |
Molecular Formula |
C15H15N | |
Source | PubChem | |
URL | https://pubchem.ncbi.nlm.nih.gov | |
Description | Data deposited in or computed by PubChem | |
DSSTOX Substance ID |
DTXSID4064185 | |
Record name | Acridine, 9,10-dihydro-9,9-dimethyl- | |
Source | EPA DSSTox | |
URL | https://comptox.epa.gov/dashboard/DTXSID4064185 | |
Description | DSSTox provides a high quality public chemistry resource for supporting improved predictive toxicology. | |
Molecular Weight |
209.29 g/mol | |
Source | PubChem | |
URL | https://pubchem.ncbi.nlm.nih.gov | |
Description | Data deposited in or computed by PubChem | |
CAS No. |
6267-02-3 | |
Record name | 9,9-Dimethyl-9,10-dihydroacridine | |
Source | CAS Common Chemistry | |
URL | https://commonchemistry.cas.org/detail?cas_rn=6267-02-3 | |
Description | CAS Common Chemistry is an open community resource for accessing chemical information. Nearly 500,000 chemical substances from CAS REGISTRY cover areas of community interest, including common and frequently regulated chemicals, and those relevant to high school and undergraduate chemistry classes. This chemical information, curated by our expert scientists, is provided in alignment with our mission as a division of the American Chemical Society. | |
Explanation | The data from CAS Common Chemistry is provided under a CC-BY-NC 4.0 license, unless otherwise stated. | |
Record name | 9,9-Dimethylcarbazine | |
Source | ChemIDplus | |
URL | https://pubchem.ncbi.nlm.nih.gov/substance/?source=chemidplus&sourceid=0006267023 | |
Description | ChemIDplus is a free, web search system that provides access to the structure and nomenclature authority files used for the identification of chemical substances cited in National Library of Medicine (NLM) databases, including the TOXNET system. | |
Record name | 9,9-DIMETHYLACRIDAN | |
Source | DTP/NCI | |
URL | https://dtp.cancer.gov/dtpstandard/servlet/dwindex?searchtype=NSC&outputformat=html&searchlist=36671 | |
Description | The NCI Development Therapeutics Program (DTP) provides services and resources to the academic and private-sector research communities worldwide to facilitate the discovery and development of new cancer therapeutic agents. | |
Explanation | Unless otherwise indicated, all text within NCI products is free of copyright and may be reused without our permission. Credit the National Cancer Institute as the source. | |
Record name | Acridine, 9,10-dihydro-9,9-dimethyl- | |
Source | EPA Chemicals under the TSCA | |
URL | https://www.epa.gov/chemicals-under-tsca | |
Description | EPA Chemicals under the Toxic Substances Control Act (TSCA) collection contains information on chemicals and their regulations under TSCA, including non-confidential content from the TSCA Chemical Substance Inventory and Chemical Data Reporting. | |
Record name | Acridine, 9,10-dihydro-9,9-dimethyl- | |
Source | EPA DSSTox | |
URL | https://comptox.epa.gov/dashboard/DTXSID4064185 | |
Description | DSSTox provides a high quality public chemistry resource for supporting improved predictive toxicology. | |
Record name | 9,10-dihydro-9,9-dimethylacridine | |
Source | European Chemicals Agency (ECHA) | |
URL | https://echa.europa.eu/substance-information/-/substanceinfo/100.025.852 | |
Description | The European Chemicals Agency (ECHA) is an agency of the European Union which is the driving force among regulatory authorities in implementing the EU's groundbreaking chemicals legislation for the benefit of human health and the environment as well as for innovation and competitiveness. | |
Explanation | Use of the information, documents and data from the ECHA website is subject to the terms and conditions of this Legal Notice, and subject to other binding limitations provided for under applicable law, the information, documents and data made available on the ECHA website may be reproduced, distributed and/or used, totally or in part, for non-commercial purposes provided that ECHA is acknowledged as the source: "Source: European Chemicals Agency, http://echa.europa.eu/". Such acknowledgement must be included in each copy of the material. ECHA permits and encourages organisations and individuals to create links to the ECHA website under the following cumulative conditions: Links can only be made to webpages that provide a link to the Legal Notice page. | |
Record name | 9,9-DIMETHYLCARBAZINE | |
Source | FDA Global Substance Registration System (GSRS) | |
URL | https://gsrs.ncats.nih.gov/ginas/app/beta/substances/TOD9JH3PHC | |
Description | The FDA Global Substance Registration System (GSRS) enables the efficient and accurate exchange of information on what substances are in regulated products. Instead of relying on names, which vary across regulatory domains, countries, and regions, the GSRS knowledge base makes it possible for substances to be defined by standardized, scientific descriptions. | |
Explanation | Unless otherwise noted, the contents of the FDA website (www.fda.gov), both text and graphics, are not copyrighted. They are in the public domain and may be republished, reprinted and otherwise used freely by anyone without the need to obtain permission from FDA. Credit to the U.S. Food and Drug Administration as the source is appreciated but not required. | |
Retrosynthesis Analysis
AI-Powered Synthesis Planning: Our tool employs the Template_relevance Pistachio, Template_relevance Bkms_metabolic, Template_relevance Pistachio_ringbreaker, Template_relevance Reaxys, Template_relevance Reaxys_biocatalysis model, leveraging a vast database of chemical reactions to predict feasible synthetic routes.
One-Step Synthesis Focus: Specifically designed for one-step synthesis, it provides concise and direct routes for your target compounds, streamlining the synthesis process.
Accurate Predictions: Utilizing the extensive PISTACHIO, BKMS_METABOLIC, PISTACHIO_RINGBREAKER, REAXYS, REAXYS_BIOCATALYSIS database, our tool offers high-accuracy predictions, reflecting the latest in chemical research and data.
Strategy Settings
Precursor scoring | Relevance Heuristic |
---|---|
Min. plausibility | 0.01 |
Model | Template_relevance |
Template Set | Pistachio/Bkms_metabolic/Pistachio_ringbreaker/Reaxys/Reaxys_biocatalysis |
Top-N result to add to graph | 6 |
Feasible Synthetic Routes
Q1: What is the molecular formula and weight of 9,9-Dimethyl-9,10-dihydroacridine?
A1: The molecular formula of 9,9-Dimethyl-9,10-dihydroacridine is C15H15N, and its molecular weight is 209.29 g/mol.
Q2: Are there any characteristic spectroscopic features of DMAC?
A2: DMAC derivatives often exhibit characteristic absorption and emission spectra, which can be influenced by the attached acceptor unit and solvent polarity [, , ]. Theoretical calculations like density functional theory (DFT) and time-dependent DFT (TD-DFT) are commonly used to analyze and predict these spectroscopic properties [, , , ].
Q3: Is DMAC thermally stable?
A3: DMAC derivatives generally show high thermal stability. For example, compounds incorporating DMAC as a donor unit have demonstrated 5% weight loss temperatures exceeding 300 °C [].
Q4: How does DMAC perform in thin films for OLED applications?
A4: DMAC derivatives have shown promising results as emitters in organic light-emitting diodes (OLEDs). They can be used in both doped and non-doped configurations [, , , , ]. Factors like molecular configuration and aggregation-induced emission (AIE) properties significantly influence the performance in thin films [, , ].
Q5: Are there any strategies to improve the stability of DMAC-based OLEDs?
A5: Research indicates that reducing the excited-state dipole moment of DMAC-based TADF emitters can improve device stability. For example, attaching a donor and acceptor at the 1- and N-positions of carbazole, respectively, resulted in a smaller excited-state dipole moment and enhanced operational lifetime compared to the 3- and N-substituted analog [].
Q6: How is computational chemistry employed in DMAC research?
A6: Computational methods like DFT and TD-DFT are extensively used to predict the electronic and optical properties of DMAC-based compounds. These calculations provide insights into energy levels, singlet-triplet energy gaps (ΔEST), and oscillator strengths, which are crucial for designing efficient TADF emitters [, , , ].
Q7: How does modifying the structure of DMAC affect its properties?
A7: Structural modifications of DMAC significantly impact its photophysical and electronic properties. For instance, introducing electron-withdrawing groups like fluorine or trifluoromethyl groups at the 2,7-positions of DMAC can fine-tune the donor strength and emission color of the resulting TADF emitters []. Similarly, changing the linking position of DMAC on a diphenylsulfone acceptor unit can influence the energy levels, minimize reorganization energy, and affect the emission color [].
Q8: How does the choice of acceptor unit impact the performance of DMAC-based TADF emitters?
A8: The acceptor unit plays a crucial role in determining the emission color and efficiency of DMAC-based TADF emitters. For example, using 2,3-dicyanopyrazino phenanthrene as an acceptor with DMAC yielded yellow to deep-red emissions, while diphenylethyne as an acceptor resulted in sky-blue emission [, ].
Q9: What is the impact of intramolecular hydrogen bonding in DMAC-based TADF emitters?
A9: Introducing intramolecular hydrogen bonding in DMAC-based TADF emitters can be beneficial. It can lead to reduced singlet-triplet energy splitting, suppressed non-radiative decay, and enhanced luminescence efficiency, ultimately improving the performance of solution-processed non-doped OLEDs [].
試験管内研究製品の免責事項と情報
BenchChemで提示されるすべての記事および製品情報は、情報提供を目的としています。BenchChemで購入可能な製品は、生体外研究のために特別に設計されています。生体外研究は、ラテン語の "in glass" に由来し、生物体の外で行われる実験を指します。これらの製品は医薬品または薬として分類されておらず、FDAから任何の医療状態、病気、または疾患の予防、治療、または治癒のために承認されていません。これらの製品を人間または動物に体内に導入する形態は、法律により厳格に禁止されています。これらのガイドラインに従うことは、研究と実験において法的および倫理的な基準の遵守を確実にするために重要です。