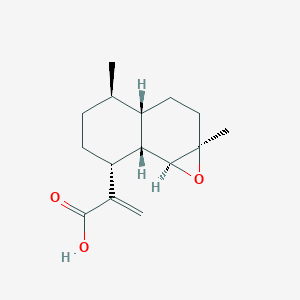
4,5-Epoxyartemisinic acid
説明
Synthesis Analysis
The synthesis of epoxy derivatives, including methods that could theoretically apply to 4,5-epoxyartemisinic acid, has been explored through various chemical strategies. For instance, the novel synthesis of epoxy derivatives from quinic and shikimic acids demonstrates the use of regio- and stereoselective epoxide ring opening, providing a template for synthesizing structurally complex epoxy compounds (Sánchez-Abella et al., 2006). Additionally, epoxy-α-ionone was synthesized using hydrogen peroxide as an oxidant, showcasing a method for obtaining epoxy compounds that might be relevant to the synthesis of this compound (Jun-ping, 2009).
Molecular Structure Analysis
Molecular structure analysis is crucial for understanding the behavior and reactivity of compounds like this compound. The study on optically active epoxy derivatives illustrates the significance of stereochemistry in determining the properties of molecules, including their reactivity and interaction with biological systems (Uebelhart et al., 1986).
Chemical Reactions and Properties
Epoxy compounds participate in a variety of chemical reactions, offering pathways to diverse derivatives. The addition reactions of 4,5-epoxy-2-hexenoic acid esters, for example, demonstrate how alcohols and diazomethane can react with epoxy compounds to form products with retained epoxy rings or transformed structures, highlighting the versatility and reactivity of epoxy groups (Glushko et al., 1982).
Physical Properties Analysis
The physical properties of epoxy compounds, including this compound, can be inferred from studies on related substances. The synthesis and identification of epoxy derivatives of 5-methylhexahydroisoindole-1,3-dione, for example, required spectrometric methods for structural elucidation, reflecting the importance of analytical techniques in characterizing the physical properties of complex organic molecules (Torrent & Alvarenga, 2021).
Chemical Properties Analysis
The chemical properties of epoxy-containing compounds, such as reactivity, stability, and interactions with other molecules, are central to their utility in synthetic chemistry and potential biological applications. Research on bio-based epoxy resins derived from furandicarboxylic acid showcases the development of materials with desirable chemical and physical properties, pointing towards the potential for eco-friendly alternatives to traditional epoxy resins (Deng et al., 2015).
科学的研究の応用
Rearrangement and Synthesis of Derivatives : A study by Rosselli et al. (2010) focused on the acid-induced rearrangement of 4,5-epoxy derivatives, leading to the synthesis of guaianes, which have potential applications in organic chemistry and possibly in pharmacology (Rosselli et al., 2010).
Pesticide Analysis : Another line of research includes the analysis of pesticides like 2,4-D herbicide, where 4,5-epoxy derivatives may play a role in the understanding of toxicology and environmental impact of such compounds. This was explored in studies by Zuanazzi et al. (2020) and others, highlighting the global trends in 2,4-D research and its ecological and health-related implications (Zuanazzi et al., 2020).
Adsorption and Environmental Applications : Khan and Akhtar (2011) investigated the adsorption behavior of 2,4,5-trichlorophenoxy acetic acid on poly-o-toluidine Zr(IV) phosphate, a study relevant to understanding how 4,5-epoxy derivatives might interact with other chemicals in environmental contexts (Khan & Akhtar, 2011).
Biochemical Pharmacology : Research by Wiśniewska et al. (2012) on a novel imidazo[1,2-a]pyridine based compound (EP6) for inhibiting 5-lipoxygenase activity demonstrates the potential biochemical applications of 4,5-epoxy derivatives in developing new pharmaceuticals (Wiśniewska et al., 2012).
Potential in Agrochemicals : The research on 4-Hydroxyphenylpyruvate Dioxygenase Inhibitors by Ndikuryayo et al. (2017) indicates a promising area for the development of innovative herbicides, where 4,5-epoxy derivatives could play a significant role (Ndikuryayo et al., 2017).
Biotransformation Studies : Zhan et al. (2015) explored the biotransformation of artemisinin by Aspergillus niger, leading to the formation of products including 4,5-epoxy derivatives, which can be used as scaffolds for further modifications in the search for new antimalarial drugs (Zhan et al., 2015).
作用機序
Target of Action
4,5-Epoxyartemisinic acid is a natural product used for research related to life sciences . .
Result of Action
Artemisia species, from which this compound is derived, have been shown to have strong antibiotic activities against various bacteria and have strong antioxidant capacity .
将来の方向性
The future directions for research on 4,5-Epoxyartemisinic acid could involve further exploration of its biosynthesis, its potential medicinal uses, and its active constituents . Additionally, synthetic approaches for endo-selective intramolecular epoxide ring opening (IERO) of 4,5-epoxy-alcohols and their applications in natural product synthesis could be investigated .
生化学分析
Biochemical Properties
4,5-Epoxyartemisinic acid plays a crucial role in biochemical reactions, particularly in the biosynthesis of artemisinin. It interacts with several enzymes and proteins during its conversion to artemisinin. One of the key enzymes involved is cytochrome P450 monooxygenase (CYP71AV1), which catalyzes the oxidation of amorpha-4,11-diene to artemisinic acid and subsequently to this compound . This interaction is essential for the formation of the endoperoxide bridge, a critical structural feature of artemisinin. Additionally, this compound interacts with other biomolecules such as NADPH and oxygen, which are required for the enzymatic reactions .
Cellular Effects
This compound has been shown to influence various cellular processes. It affects cell signaling pathways, gene expression, and cellular metabolism. Studies have demonstrated that this compound can induce apoptosis in cancer cells by generating reactive oxygen species (ROS) and disrupting mitochondrial function . This compound also modulates the expression of genes involved in cell cycle regulation and apoptosis, leading to cell cycle arrest and programmed cell death . Furthermore, this compound has been found to inhibit angiogenesis, thereby preventing the formation of new blood vessels that supply nutrients to tumors .
Molecular Mechanism
The molecular mechanism of this compound involves several key interactions at the molecular level. The compound exerts its effects by binding to specific biomolecules and enzymes, leading to their inhibition or activation. For instance, this compound binds to cytochrome P450 monooxygenase (CYP71AV1), facilitating the oxidation of amorpha-4,11-diene to artemisinic acid . This binding interaction is crucial for the formation of the endoperoxide bridge in artemisinin. Additionally, this compound can generate ROS, which can cause oxidative damage to cellular components and trigger apoptosis . The compound also influences gene expression by modulating transcription factors and signaling pathways involved in cell cycle regulation and apoptosis .
Temporal Effects in Laboratory Settings
In laboratory settings, the effects of this compound have been observed to change over time. The compound’s stability and degradation play a significant role in its long-term effects on cellular function. Studies have shown that this compound is relatively stable when stored at -20°C for up to three years in powder form . In solution, its stability decreases, and it should be stored at -80°C for optimal preservation . Over time, the compound may degrade, leading to a reduction in its efficacy and potency. Long-term exposure to this compound in in vitro and in vivo studies has shown that it can induce sustained changes in cellular function, including prolonged inhibition of cell proliferation and induction of apoptosis .
Dosage Effects in Animal Models
The effects of this compound vary with different dosages in animal models. Studies have demonstrated that low to moderate doses of the compound can effectively inhibit tumor growth and induce apoptosis without causing significant toxicity . At high doses, this compound may exhibit toxic effects, including hepatotoxicity and nephrotoxicity . These adverse effects are dose-dependent and highlight the importance of determining the optimal dosage for therapeutic applications. Additionally, threshold effects have been observed, where a minimum concentration of this compound is required to achieve significant biological effects .
Metabolic Pathways
This compound is involved in several metabolic pathways, primarily related to the biosynthesis of artemisinin. The compound is synthesized from amorpha-4,11-diene through a series of enzymatic reactions catalyzed by cytochrome P450 monooxygenase (CYP71AV1) . This pathway involves the oxidation of amorpha-4,11-diene to artemisinic acid, followed by the formation of this compound . The metabolic flux through this pathway is regulated by the availability of substrates and cofactors, such as NADPH and oxygen . Additionally, this compound can influence the levels of other metabolites in the pathway, affecting the overall production of artemisinin .
Transport and Distribution
The transport and distribution of this compound within cells and tissues are mediated by specific transporters and binding proteins. The compound is transported across cellular membranes by passive diffusion and active transport mechanisms . Once inside the cell, this compound can bind to intracellular proteins and enzymes, facilitating its distribution to various cellular compartments . The localization and accumulation of this compound within cells are influenced by factors such as pH, temperature, and the presence of binding proteins . These factors play a crucial role in determining the compound’s bioavailability and efficacy.
Subcellular Localization
The subcellular localization of this compound is essential for its activity and function. The compound is primarily localized in the endoplasmic reticulum (ER) and mitochondria, where it interacts with specific enzymes and proteins . The targeting of this compound to these organelles is mediated by post-translational modifications and targeting signals . For instance, the presence of specific amino acid sequences in the compound’s structure can direct it to the ER or mitochondria . The subcellular localization of this compound is critical for its role in biochemical reactions and cellular processes, as it ensures the compound’s proximity to its target biomolecules.
特性
IUPAC Name |
2-[(1aR,3aS,4R,7R,7aS,7bS)-1a,4-dimethyl-3,3a,4,5,6,7,7a,7b-octahydro-2H-naphtho[1,2-b]oxiren-7-yl]prop-2-enoic acid | |
---|---|---|
Source | PubChem | |
URL | https://pubchem.ncbi.nlm.nih.gov | |
Description | Data deposited in or computed by PubChem | |
InChI |
InChI=1S/C15H22O3/c1-8-4-5-11(9(2)14(16)17)12-10(8)6-7-15(3)13(12)18-15/h8,10-13H,2,4-7H2,1,3H3,(H,16,17)/t8-,10+,11+,12+,13+,15-/m1/s1 | |
Source | PubChem | |
URL | https://pubchem.ncbi.nlm.nih.gov | |
Description | Data deposited in or computed by PubChem | |
InChI Key |
CQNNGKUJKJXDCO-IEFGFUDGSA-N | |
Source | PubChem | |
URL | https://pubchem.ncbi.nlm.nih.gov | |
Description | Data deposited in or computed by PubChem | |
Canonical SMILES |
CC1CCC(C2C1CCC3(C2O3)C)C(=C)C(=O)O | |
Source | PubChem | |
URL | https://pubchem.ncbi.nlm.nih.gov | |
Description | Data deposited in or computed by PubChem | |
Isomeric SMILES |
C[C@@H]1CC[C@H]([C@@H]2[C@H]1CC[C@@]3([C@H]2O3)C)C(=C)C(=O)O | |
Source | PubChem | |
URL | https://pubchem.ncbi.nlm.nih.gov | |
Description | Data deposited in or computed by PubChem | |
Molecular Formula |
C15H22O3 | |
Source | PubChem | |
URL | https://pubchem.ncbi.nlm.nih.gov | |
Description | Data deposited in or computed by PubChem | |
Molecular Weight |
250.33 g/mol | |
Source | PubChem | |
URL | https://pubchem.ncbi.nlm.nih.gov | |
Description | Data deposited in or computed by PubChem | |
Retrosynthesis Analysis
AI-Powered Synthesis Planning: Our tool employs the Template_relevance Pistachio, Template_relevance Bkms_metabolic, Template_relevance Pistachio_ringbreaker, Template_relevance Reaxys, Template_relevance Reaxys_biocatalysis model, leveraging a vast database of chemical reactions to predict feasible synthetic routes.
One-Step Synthesis Focus: Specifically designed for one-step synthesis, it provides concise and direct routes for your target compounds, streamlining the synthesis process.
Accurate Predictions: Utilizing the extensive PISTACHIO, BKMS_METABOLIC, PISTACHIO_RINGBREAKER, REAXYS, REAXYS_BIOCATALYSIS database, our tool offers high-accuracy predictions, reflecting the latest in chemical research and data.
Strategy Settings
Precursor scoring | Relevance Heuristic |
---|---|
Min. plausibility | 0.01 |
Model | Template_relevance |
Template Set | Pistachio/Bkms_metabolic/Pistachio_ringbreaker/Reaxys/Reaxys_biocatalysis |
Top-N result to add to graph | 6 |
Feasible Synthetic Routes
試験管内研究製品の免責事項と情報
BenchChemで提示されるすべての記事および製品情報は、情報提供を目的としています。BenchChemで購入可能な製品は、生体外研究のために特別に設計されています。生体外研究は、ラテン語の "in glass" に由来し、生物体の外で行われる実験を指します。これらの製品は医薬品または薬として分類されておらず、FDAから任何の医療状態、病気、または疾患の予防、治療、または治癒のために承認されていません。これらの製品を人間または動物に体内に導入する形態は、法律により厳格に禁止されています。これらのガイドラインに従うことは、研究と実験において法的および倫理的な基準の遵守を確実にするために重要です。