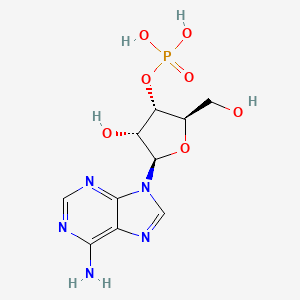
3'-Adenylic acid
Vue d'ensemble
Description
L’acide adénylique 3’, également connu sous le nom d’adénosine 3’-monophosphate, est un nucléotide qui joue un rôle crucial dans divers processus biologiques. Il est constitué d’un groupe phosphate, du sucre ribose et de la base azotée adénine. Ce composé est un ester de l’acide phosphorique et du nucléoside adénosine .
Applications De Recherche Scientifique
L’acide adénylique 3’ a un large éventail d’applications en recherche scientifique :
Chimie : Il est utilisé comme précurseur dans la synthèse d’autres nucléotides et d’analogues de nucléotides.
Biologie : Il joue un rôle dans les voies de signalisation cellulaire et est impliqué dans la régulation de divers processus métaboliques.
Médecine : L’acide adénylique 3’ est étudié pour ses applications thérapeutiques potentielles, y compris son rôle dans la modulation immunitaire et en tant que traitement potentiel pour certains troubles métaboliques.
Industrie : Il est utilisé dans la production de produits à base de nucléotides, tels que les compléments alimentaires et les produits pharmaceutiques
Mécanisme D'action
L’acide adénylique 3’ exerce ses effets principalement par son rôle de nucléotide. Il peut être converti en d’autres nucléotides, tels que l’adénosine triphosphate (ATP) et l’adénosine diphosphate (ADP), qui sont essentiels pour le transfert et le stockage de l’énergie dans les cellules. De plus, il peut agir comme une molécule de signalisation, influençant divers processus cellulaires en activant des enzymes et des récepteurs spécifiques .
Composés similaires :
Adénosine 5’-monophosphate : Structure similaire mais avec le groupe phosphate attaché à la position 5’.
Inosine monophosphate : Contient de l’hypoxanthine au lieu de l’adénine.
Guanosine monophosphate : Contient de la guanine au lieu de l’adénine.
Unicité : L’acide adénylique 3’ est unique en raison du positionnement spécifique du groupe phosphate à la position 3’, ce qui influence ses propriétés biochimiques et ses interactions. Cette structure distincte lui permet de participer à des réactions enzymatiques spécifiques et à des voies de signalisation qui ne sont pas accessibles à ses analogues .
Safety and Hazards
Orientations Futures
The dynamic features of these machineries play important roles in regulating polyadenylation and deadenylation . The accurate formation of 3′ end on newly synthesized mRNA, known as polyadenylation, is critical for mRNA maturation, mRNA stability, and mRNA export from the nucleus into the cytoplasm .
Analyse Biochimique
Biochemical Properties
3’-Adenylic acid is integral to many biochemical reactions. It is interconverted to adenosine triphosphate (ATP) and adenosine diphosphate (ADP), which are essential for energy transfer within cells . The compound interacts with enzymes such as adenylate kinase, which catalyzes the conversion of two molecules of ADP into ATP and AMP. Additionally, 3’-Adenylic acid is involved in the activation of enzymes like myophosphorylase-b, which plays a role in glycogen metabolism .
Cellular Effects
3’-Adenylic acid influences various cellular processes, including cell signaling pathways, gene expression, and cellular metabolism. It acts as a signaling molecule that can activate protein kinases, leading to the phosphorylation of target proteins and subsequent changes in cellular activities . The compound also affects gene expression by serving as a precursor for cyclic adenosine monophosphate (cAMP), a secondary messenger that regulates transcription factors and other proteins involved in gene regulation .
Molecular Mechanism
At the molecular level, 3’-Adenylic acid exerts its effects through binding interactions with various biomolecules. It can bind to and activate adenylate cyclase, an enzyme that converts ATP to cAMP . This activation leads to a cascade of intracellular events, including the activation of protein kinase A (PKA), which phosphorylates multiple target proteins, thereby modulating their activity . Additionally, 3’-Adenylic acid can be converted into inosine monophosphate by the enzyme myoadenylate deaminase, which plays a role in purine metabolism .
Temporal Effects in Laboratory Settings
In laboratory settings, the effects of 3’-Adenylic acid can change over time due to its stability and degradation. The compound is relatively stable under physiological conditions but can be hydrolyzed by phosphodiesterases, leading to the formation of adenosine and inorganic phosphate . Long-term studies have shown that 3’-Adenylic acid can influence cellular functions such as energy metabolism and gene expression over extended periods .
Dosage Effects in Animal Models
The effects of 3’-Adenylic acid vary with different dosages in animal models. At low doses, it can enhance cellular energy levels and improve metabolic functions . At high doses, it may lead to toxic effects, including disruptions in cellular signaling pathways and metabolic imbalances . Threshold effects have been observed, where a certain dosage is required to elicit a significant biological response .
Metabolic Pathways
3’-Adenylic acid is involved in several metabolic pathways, including the purine nucleotide cycle and glycolysis . It can be converted into inosine monophosphate by myoadenylate deaminase, which is then further metabolized to uric acid for excretion . The compound also plays a role in the regulation of glycolytic enzymes, influencing metabolic flux and the levels of various metabolites .
Transport and Distribution
Within cells, 3’-Adenylic acid is transported and distributed by specific transporters and binding proteins. It is taken up by cells through nucleoside transporters and can be localized to various cellular compartments, including the cytoplasm and mitochondria . The distribution of 3’-Adenylic acid within tissues is influenced by its interactions with binding proteins that facilitate its transport and accumulation .
Subcellular Localization
3’-Adenylic acid is localized to specific subcellular compartments, where it exerts its biological functions. It is found in the cytoplasm, where it participates in energy metabolism and signaling pathways . Additionally, it can be targeted to the mitochondria, where it plays a role in oxidative phosphorylation and ATP synthesis . The localization of 3’-Adenylic acid is regulated by targeting signals and post-translational modifications that direct it to specific organelles .
Méthodes De Préparation
Voies de synthèse et conditions de réaction : L’acide adénylique 3’ peut être synthétisé par phosphorylation de l’adénosine. La réaction implique généralement l’utilisation d’agents phosphorylants tels que l’oxychlorure de phosphore (POCl3) en présence d’une base comme la pyridine. Les conditions réactionnelles nécessitent souvent des solvants anhydres et des températures contrôlées pour assurer la formation sélective de l’ester phosphate 3’ .
Méthodes de production industrielle : En milieu industriel, l’acide adénylique 3’ est souvent produit par des méthodes enzymatiques. Des enzymes telles que l’adénosine kinase peuvent catalyser la phosphorylation de l’adénosine pour former l’acide adénylique 3’. Cette méthode est préférée en raison de sa spécificité et de son efficacité .
Types de réactions :
Oxydation : L’acide adénylique 3’ peut subir des réactions d’oxydation, en particulier au niveau de la fraction adénine. Les oxydants courants comprennent le peroxyde d’hydrogène et le permanganate de potassium.
Réduction : Les réactions de réduction sont moins fréquentes mais peuvent se produire dans des conditions spécifiques en utilisant des agents réducteurs tels que le borohydrure de sodium.
Réactifs et conditions courants :
Oxydation : Peroxyde d’hydrogène, permanganate de potassium.
Réduction : Borohydrure de sodium.
Substitution : Divers nucléophiles en milieu acide ou basique.
Principaux produits :
Oxydation : Dérivés oxydés de l’adénine.
Réduction : Formes réduites de la fraction ribose ou adénine.
Substitution : Analogues de nucléotides avec des groupes phosphate modifiés.
Comparaison Avec Des Composés Similaires
Adenosine 5’-monophosphate: Similar in structure but with the phosphate group attached at the 5’ position.
Inosine monophosphate: Contains hypoxanthine instead of adenine.
Guanosine monophosphate: Contains guanine instead of adenine.
Uniqueness: 3’-Adenylic acid is unique due to the specific positioning of the phosphate group at the 3’ position, which influences its biochemical properties and interactions. This distinct structure allows it to participate in specific enzymatic reactions and signaling pathways that are not accessible to its analogs .
Propriétés
IUPAC Name |
[5-(6-aminopurin-9-yl)-4-hydroxy-2-(hydroxymethyl)oxolan-3-yl] dihydrogen phosphate | |
---|---|---|
Details | Computed by Lexichem TK 2.7.0 (PubChem release 2021.05.07) | |
Source | PubChem | |
URL | https://pubchem.ncbi.nlm.nih.gov | |
Description | Data deposited in or computed by PubChem | |
InChI |
InChI=1S/C10H14N5O7P/c11-8-5-9(13-2-12-8)15(3-14-5)10-6(17)7(4(1-16)21-10)22-23(18,19)20/h2-4,6-7,10,16-17H,1H2,(H2,11,12,13)(H2,18,19,20) | |
Details | Computed by InChI 1.0.6 (PubChem release 2021.05.07) | |
Source | PubChem | |
URL | https://pubchem.ncbi.nlm.nih.gov | |
Description | Data deposited in or computed by PubChem | |
InChI Key |
LNQVTSROQXJCDD-UHFFFAOYSA-N | |
Details | Computed by InChI 1.0.6 (PubChem release 2021.05.07) | |
Source | PubChem | |
URL | https://pubchem.ncbi.nlm.nih.gov | |
Description | Data deposited in or computed by PubChem | |
Canonical SMILES |
C1=NC(=C2C(=N1)N(C=N2)C3C(C(C(O3)CO)OP(=O)(O)O)O)N | |
Details | Computed by OEChem 2.3.0 (PubChem release 2021.05.07) | |
Source | PubChem | |
URL | https://pubchem.ncbi.nlm.nih.gov | |
Description | Data deposited in or computed by PubChem | |
Molecular Formula |
C10H14N5O7P | |
Details | Computed by PubChem 2.1 (PubChem release 2021.05.07) | |
Source | PubChem | |
URL | https://pubchem.ncbi.nlm.nih.gov | |
Description | Data deposited in or computed by PubChem | |
Molecular Weight |
347.22 g/mol | |
Details | Computed by PubChem 2.1 (PubChem release 2021.05.07) | |
Source | PubChem | |
URL | https://pubchem.ncbi.nlm.nih.gov | |
Description | Data deposited in or computed by PubChem | |
CAS No. |
84-21-9 | |
Record name | 3'-Adenylic acid | |
Source | DTP/NCI | |
URL | https://dtp.cancer.gov/dtpstandard/servlet/dwindex?searchtype=NSC&outputformat=html&searchlist=210570 | |
Description | The NCI Development Therapeutics Program (DTP) provides services and resources to the academic and private-sector research communities worldwide to facilitate the discovery and development of new cancer therapeutic agents. | |
Explanation | Unless otherwise indicated, all text within NCI products is free of copyright and may be reused without our permission. Credit the National Cancer Institute as the source. | |
Q1: What are the main biological effects of 3'-adenylic acid on cell division and the mitotic cycle?
A1: Research suggests that this compound exhibits an inhibitory effect on cell division. [] This inhibition is linked to a significant increase in the duration of the mitotic cycle, as observed in Vicia faba. [] It's proposed that the compound's strong influence on the nucleus and chromosomes, particularly the induction of picnosis, stems from its potential to cause DNA depolymerization. [] This disruption of DNA integrity could further impede the synthesis of macromolecules. []
Q2: What are the key differences in this compound content observed in various marine organisms, and what enzymatic basis explains these variations?
A2: Significant variations in this compound levels are observed across different marine species. For instance, salted clam and cuttlefish pickles exhibit high this compound concentrations compared to other seafood pickles. [] This difference is attributed to the absence of adenylic deaminase in clams and certain invertebrates, leading to the accumulation of adenylic acid and classifying them as AMP-type organisms. [] In contrast, salted yellow tail pickles contain lower levels of this compound but significant amounts of 5'-inosinic acid, characterizing them as IMP-type. [] This difference highlights the diverse metabolic pathways and enzyme activities present in various marine organisms.
Q3: How does the presence of this compound contribute to the distinct flavor profiles of certain foods?
A3: this compound, along with other nucleotides and amino acids, plays a crucial role in shaping the unique flavor profiles of various foods. For example, the characteristic flavor of salted oyster pickles is attributed to a high concentration of this compound and the presence of sweet amino acids like lysine, threonine, isoleucine, and leucine. [] Additionally, the combined effect of this compound with glutamic acid and aspartic acid contributes to the specific taste of salted yellow tail pickles, demonstrating a potential synergistic interaction between these flavor components. []
Q4: Can you elaborate on the metabolic pathways affected by sodium fluoride (NaF) stress in silkworms, particularly those involving this compound?
A4: Metabolomic analysis of silkworm midgut tissue exposed to NaF stress revealed significant alterations in purine metabolism, particularly in fluoride-tolerant strains. [] A notable observation was the upregulation of this compound and hypoxanthine, alongside the downregulation of guanine, allantoic acid, xanthine, N-acetyl-L-glutamic acid, and pyruvate. [] These findings suggest a potential link between these metabolic pathways, including those involving this compound, and the mechanisms underlying NaF tolerance in silkworms.
Q5: What is the role of microbial ribonucleases in the context of this compound?
A5: Microbial ribonucleases, specifically those exhibiting this compound specificity, like RNase T2 from Aspergillus oryzae, play a crucial role in RNA degradation by cleaving phosphodiester bonds adjacent to this compound residues. [, ] This degradation process is essential for generating mature, functional RNA molecules from their precursors. [] The discovery and characterization of these enzymes highlight the importance of microbial sources in understanding RNA metabolism and the diverse roles of ribonucleases in biological systems.
Avertissement et informations sur les produits de recherche in vitro
Veuillez noter que tous les articles et informations sur les produits présentés sur BenchChem sont destinés uniquement à des fins informatives. Les produits disponibles à l'achat sur BenchChem sont spécifiquement conçus pour des études in vitro, qui sont réalisées en dehors des organismes vivants. Les études in vitro, dérivées du terme latin "in verre", impliquent des expériences réalisées dans des environnements de laboratoire contrôlés à l'aide de cellules ou de tissus. Il est important de noter que ces produits ne sont pas classés comme médicaments et n'ont pas reçu l'approbation de la FDA pour la prévention, le traitement ou la guérison de toute condition médicale, affection ou maladie. Nous devons souligner que toute forme d'introduction corporelle de ces produits chez les humains ou les animaux est strictement interdite par la loi. Il est essentiel de respecter ces directives pour assurer la conformité aux normes légales et éthiques en matière de recherche et d'expérimentation.