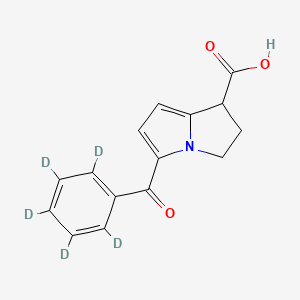
Ketorolac-d5
Übersicht
Beschreibung
Ketorolac-d5 is the labeled analogue of Ketorolac . Ketorolac is a non-steroidal anti-inflammatory drug (NSAID) and a nonselective COX inhibitor . It is used to relieve moderately severe pain, usually pain that occurs after an operation or other painful procedure . It is not a narcotic and is not habit-forming .
Synthesis Analysis
Ketorolac-d5 is intended for use as an internal standard for the quantification of ketorolac by GC- or LC-MS . The inherent stability and biochemical interaction of Ketorolac and its degradation products have been studied on the basis of quantum mechanical approaches . Density functional theory (DFT) with B3LYP/ 6-31G (d) has been employed to optimize the structures .Molecular Structure Analysis
The molecular formula of Ketorolac-d5 is C15H13NO3 . The molecular weight is 260.30 g/mol . The structure of Ketorolac-d5 has been optimized using Density functional theory (DFT) with B3LYP/ 6-31G (d) .Chemical Reactions Analysis
Ketorolac-d5, like other NSAIDs, blocks cyclooxygenases (COX), which are enzymes that convert arachidonic acid into prostaglandins . The inherent stability and biochemical interaction of Ketorolac and its degradation products have been studied on the basis of quantum mechanical approaches .Physical And Chemical Properties Analysis
The molecular weight of Ketorolac-d5 is 260.30 g/mol . It has a Hydrogen Bond Donor Count of 1 and a Hydrogen Bond Acceptor Count of 3 . The Rotatable Bond Count is 3 . The Exact Mass is 260.120927006 g/mol and the Monoisotopic Mass is 260.120927006 g/mol .Wissenschaftliche Forschungsanwendungen
Controlled Drug Release Systems
Ketorolac tromethamine is a non-steroidal anti-inflammatory drug used in the management of severe pain . However, its half-life is within the range of 2.5–4 hours, which means repeated doses are needed in a day to maintain the therapeutic level . To address this, researchers have developed pH-responsive hydrogels for the controlled release of Ketorolac tromethamine . These hydrogels were developed by the free radical polymerization technique .
Pain Management
Ketorolac tromethamine is widely used for the management of severe pain . It is a non-steroidal anti-inflammatory drug (NSAID) with low anti-inflammatory and high analgesic activity . This makes it an important tool in pain management research.
Formulation of Orally Disintegrating Tablets
Orally disintegrating tablets of Ketorolac tromethamine have been prepared by direct compression using various super disintegrants . These tablets dissolve in the oral cavity within 1 minute of contact with saliva, increasing the effectiveness of the active pharmaceutical ingredient without the need for water for administration . This research has implications for improving patient compliance, especially in geriatric and pediatric patients.
Environmental Research
Ketorolac has also been used in environmental research. For instance, studies have been conducted on the removal of Ketorolac through photoelectrocatalysis using TiO2 nanotubes in water systems . This research is important for understanding the environmental impact of pharmaceuticals and developing methods for their removal from water systems.
Wirkmechanismus
Target of Action
Ketorolac-d5, like its parent compound Ketorolac, is a non-steroidal anti-inflammatory drug (NSAID) that primarily targets the cyclooxygenase enzymes (COX-1 and COX-2) . These enzymes play a crucial role in the synthesis of prostaglandins, which are key mediators of inflammation, pain, and fever .
Mode of Action
Ketorolac-d5 acts by competitively inhibiting the COX enzymes, thereby blocking the conversion of arachidonic acid to prostaglandins . Although it is non-selective and inhibits both COX-1 and COX-2 enzymes, its clinical efficacy is derived from its COX-2 inhibition . The COX-2 enzyme is inducible and is responsible for the production of prostaglandins that mediate pain and inflammation .
Biochemical Pathways
By inhibiting the COX enzymes, Ketorolac-d5 disrupts the synthesis of prostaglandins, thereby reducing inflammation, pain, and fever . Prostaglandins are involved in various physiological processes, including the regulation of inflammation, pain perception, and body temperature. Therefore, the inhibition of prostaglandin synthesis by Ketorolac-d5 can lead to a reduction in these responses .
Pharmacokinetics
Ketorolac is administered as the tromethamine salt orally, intramuscularly, intravenously, and as a topical ophthalmic solution . It has a high bioavailability, with 80-100% for oral administration and 100% for intravenous/intramuscular administration . It is metabolized in the liver and has an elimination half-life ranging from 3.5 to 9.2 hours in young adults and 4.7 to 8.6 hours in the elderly . The majority of the drug is excreted by the kidneys .
Result of Action
The primary result of Ketorolac-d5’s action is the reduction of inflammation, pain, and fever . By inhibiting the synthesis of prostaglandins, it reduces the physiological responses mediated by these compounds. This makes it a useful tool in the management of conditions such as postoperative pain, rheumatoid arthritis, osteoarthritis, menstrual disorders, and headaches .
Action Environment
The action of Ketorolac-d5 can be influenced by various environmental factors. For instance, the presence of other drugs can affect its metabolism and excretion, potentially altering its efficacy and side effect profile. Additionally, individual patient factors such as age, weight, renal function, and liver function can also influence the drug’s pharmacokinetics and pharmacodynamics .
Safety and Hazards
Zukünftige Richtungen
Ketorolac is used for the short-term management of moderate to severe pain . Because of the risk of serious side effects, any leftover ketorolac should not be saved for use in the future, and it should not be shared with other people . The dose of this medicine will be different for different patients .
Eigenschaften
IUPAC Name |
5-(2,3,4,5,6-pentadeuteriobenzoyl)-2,3-dihydro-1H-pyrrolizine-1-carboxylic acid | |
---|---|---|
Source | PubChem | |
URL | https://pubchem.ncbi.nlm.nih.gov | |
Description | Data deposited in or computed by PubChem | |
InChI |
InChI=1S/C15H13NO3/c17-14(10-4-2-1-3-5-10)13-7-6-12-11(15(18)19)8-9-16(12)13/h1-7,11H,8-9H2,(H,18,19)/i1D,2D,3D,4D,5D | |
Source | PubChem | |
URL | https://pubchem.ncbi.nlm.nih.gov | |
Description | Data deposited in or computed by PubChem | |
InChI Key |
OZWKMVRBQXNZKK-RALIUCGRSA-N | |
Source | PubChem | |
URL | https://pubchem.ncbi.nlm.nih.gov | |
Description | Data deposited in or computed by PubChem | |
Canonical SMILES |
C1CN2C(=CC=C2C(=O)C3=CC=CC=C3)C1C(=O)O | |
Source | PubChem | |
URL | https://pubchem.ncbi.nlm.nih.gov | |
Description | Data deposited in or computed by PubChem | |
Isomeric SMILES |
[2H]C1=C(C(=C(C(=C1[2H])[2H])C(=O)C2=CC=C3N2CCC3C(=O)O)[2H])[2H] | |
Source | PubChem | |
URL | https://pubchem.ncbi.nlm.nih.gov | |
Description | Data deposited in or computed by PubChem | |
Molecular Formula |
C15H13NO3 | |
Source | PubChem | |
URL | https://pubchem.ncbi.nlm.nih.gov | |
Description | Data deposited in or computed by PubChem | |
Molecular Weight |
260.30 g/mol | |
Source | PubChem | |
URL | https://pubchem.ncbi.nlm.nih.gov | |
Description | Data deposited in or computed by PubChem | |
Synthesis routes and methods I
Procedure details
Synthesis routes and methods II
Procedure details
Synthesis routes and methods III
Procedure details
Synthesis routes and methods IV
Procedure details
Retrosynthesis Analysis
AI-Powered Synthesis Planning: Our tool employs the Template_relevance Pistachio, Template_relevance Bkms_metabolic, Template_relevance Pistachio_ringbreaker, Template_relevance Reaxys, Template_relevance Reaxys_biocatalysis model, leveraging a vast database of chemical reactions to predict feasible synthetic routes.
One-Step Synthesis Focus: Specifically designed for one-step synthesis, it provides concise and direct routes for your target compounds, streamlining the synthesis process.
Accurate Predictions: Utilizing the extensive PISTACHIO, BKMS_METABOLIC, PISTACHIO_RINGBREAKER, REAXYS, REAXYS_BIOCATALYSIS database, our tool offers high-accuracy predictions, reflecting the latest in chemical research and data.
Strategy Settings
Precursor scoring | Relevance Heuristic |
---|---|
Min. plausibility | 0.01 |
Model | Template_relevance |
Template Set | Pistachio/Bkms_metabolic/Pistachio_ringbreaker/Reaxys/Reaxys_biocatalysis |
Top-N result to add to graph | 6 |
Feasible Synthetic Routes
Haftungsausschluss und Informationen zu In-Vitro-Forschungsprodukten
Bitte beachten Sie, dass alle Artikel und Produktinformationen, die auf BenchChem präsentiert werden, ausschließlich zu Informationszwecken bestimmt sind. Die auf BenchChem zum Kauf angebotenen Produkte sind speziell für In-vitro-Studien konzipiert, die außerhalb lebender Organismen durchgeführt werden. In-vitro-Studien, abgeleitet von dem lateinischen Begriff "in Glas", beinhalten Experimente, die in kontrollierten Laborumgebungen unter Verwendung von Zellen oder Geweben durchgeführt werden. Es ist wichtig zu beachten, dass diese Produkte nicht als Arzneimittel oder Medikamente eingestuft sind und keine Zulassung der FDA für die Vorbeugung, Behandlung oder Heilung von medizinischen Zuständen, Beschwerden oder Krankheiten erhalten haben. Wir müssen betonen, dass jede Form der körperlichen Einführung dieser Produkte in Menschen oder Tiere gesetzlich strikt untersagt ist. Es ist unerlässlich, sich an diese Richtlinien zu halten, um die Einhaltung rechtlicher und ethischer Standards in Forschung und Experiment zu gewährleisten.