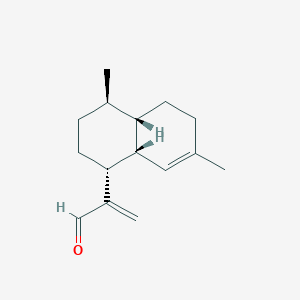
Artemisinic aldehyde
Übersicht
Beschreibung
Artemisinic aldehyde is a compound with the molecular formula C15H22O . It is a key intermediate in the biosynthesis of artemisinin, a potent antimalarial drug derived from the sweet wormwood plant (Artemisia annua) .
Synthesis Analysis
The synthesis of artemisinic aldehyde involves several steps. Two molecules of IPP and one molecule of DMAPP are catalyzed by FPPS (farnesyl diphosphate synthase) to form 15-carbon FPP (farnesyl diphosphate), which enters the downstream metabolic pathway of artemisinin biosynthesis . The amorpha-4,11-diene produced is then oxidized to artemisinic alcohol and artemisinic aldehyde .Molecular Structure Analysis
Artemisinic aldehyde has a molecular weight of 218.335 Da and a mono-isotopic mass of 218.167068 Da . It has four defined stereocentres .Chemical Reactions Analysis
Artemisinic aldehyde is converted into dihydroartemisinic aldehyde by the action of artemisinic aldehyde Δ11(13) reductase . This is then oxidized by aldehyde dehydrogenase to dihydroartemisinic acid .Physical And Chemical Properties Analysis
Artemisinic aldehyde has a density of 0.9±0.1 g/cm3, a boiling point of 325.1±11.0 °C at 760 mmHg, and a vapour pressure of 0.0±0.7 mmHg at 25°C . It has a molar refractivity of 66.7±0.3 cm3 and a polar surface area of 17 Å2 .Wissenschaftliche Forschungsanwendungen
Malaria Treatment
Artemisinic aldehyde is a key compound in the biosynthesis of Artemisinin , a sesquiterpene endoperoxide lactone derived from Artemisia annua . Artemisinin is highly effective against the malaria parasite Plasmodium falciparum . It forms the core component of artemisinin-based combination therapies (ACTs), especially for the treatment of multidrug-resistant malaria .
Anticancer Activity
Artemisinin and its derivatives, which are synthesized from Artemisinic aldehyde, demonstrate anticancer activity . The exact mechanisms of this anticancer activity are still under investigation, but it opens up potential avenues for cancer treatment.
Anti-Inflammatory Properties
Artemisinin and its derivatives also exhibit anti-inflammatory properties . This suggests that Artemisinic aldehyde, as a precursor to Artemisinin, could be used in the development of anti-inflammatory drugs.
Antiviral Activity
Artemisinin and its derivatives have shown antiviral activity . This implies that Artemisinic aldehyde could potentially be used in the development of antiviral drugs.
Anti-SARS-CoV-2 Activity
Artemisinin and its derivatives have shown activity against SARS-CoV-2 . This suggests that Artemisinic aldehyde could potentially be used in the development of treatments for COVID-19.
Biosynthesis in Yeast
Artemisinic aldehyde is a key intermediate in the biosynthesis of Artemisinin. Advances in synthetic biology have enabled the production of Artemisinin in yeast . Artemisinic aldehyde is converted to dihydroartemisinic aldehyde (DHAAA) by the enzyme DBR2 . This process has been used to improve yields of Artemisinin production in yeast .
Genetic Engineering in Plants
Artemisinic aldehyde is also involved in the genetic engineering of Artemisia annua to improve the content of Artemisinin . This includes overexpressing Artemisinin biosynthesis pathway genes, blocking key genes in competitive pathways, and regulating the expression of transcription factors related to Artemisinin biosynthesis .
Biotechnological Approaches for Enhanced Biosynthesis
Various biotechnological approaches have been explored for the enhanced biosynthesis of Artemisinin and its derivatives . These approaches target the overproduction of Artemisinin and its derivatives by manipulating the biosynthetic pathway genes, trichome development genes, and rol genes .
Safety and Hazards
Zukünftige Richtungen
Wirkmechanismus
Artemisinic aldehyde, also known as artemisic aldehyde, is a key intermediate in the biosynthesis of artemisinin, a potent anti-malarial compound . This article will cover the mechanism of action of artemisinic aldehyde, including its targets, mode of action, biochemical pathways, pharmacokinetics, results of action, and the influence of environmental factors.
Target of Action
Artemisinic aldehyde primarily targets the enzymes DBR2 (artemisinic aldehyde Δ11 (13) reductase) and ALDH1 (aldehyde dehydrogenase 1) . These enzymes play a crucial role in the biosynthesis of artemisinin, a compound highly effective against the malaria parasite Plasmodium falciparum .
Mode of Action
Artemisinic aldehyde interacts with its targets, DBR2 and ALDH1, to generate dihydroartemisinic aldehyde (DHAAA) and dihydroartemisinic acid (DHAA), respectively . This interaction results in the conversion of artemisinic aldehyde into DHAAA and subsequently DHAA, which are key steps in the biosynthesis of artemisinin .
Biochemical Pathways
The biochemical pathway of artemisinin biosynthesis, in which artemisinic aldehyde plays a key role, has been completely elucidated . Artemisinic aldehyde is catalyzed by DBR2 to generate DHAAA, and DHAAA is then converted to DHAA by ALDH1 . This pathway is crucial for the production of artemisinin, a potent anti-malarial compound .
Pharmacokinetics
Information on the ADME properties (Absorption, Distribution, Metabolism, and Excretion) of artemisinic aldehyde is currently limited. It is known that artemisinic aldehyde is a lipophilic compound, which may influence its absorption and distribution .
Result of Action
The result of the action of artemisinic aldehyde is the production of DHAAA and subsequently DHAA . These compounds are precursors in the biosynthesis of artemisinin, which has potent anti-malarial activity . Additionally, artemisinin and its derivatives have demonstrated anticancer, anti-inflammation, antiviral, and anti-SARS-CoV-2 activity .
Action Environment
The action of artemisinic aldehyde can be influenced by various environmental factors. For instance, the biosynthesis of artemisinin, in which artemisinic aldehyde plays a key role, occurs in the glandular trichomes of the plant Artemisia annua . Therefore, factors that affect the growth and development of these trichomes could potentially influence the action of artemisinic aldehyde.
Eigenschaften
IUPAC Name |
2-[(1R,4R,4aS,8aR)-4,7-dimethyl-1,2,3,4,4a,5,6,8a-octahydronaphthalen-1-yl]prop-2-enal | |
---|---|---|
Source | PubChem | |
URL | https://pubchem.ncbi.nlm.nih.gov | |
Description | Data deposited in or computed by PubChem | |
InChI |
InChI=1S/C15H22O/c1-10-4-6-13-11(2)5-7-14(12(3)9-16)15(13)8-10/h8-9,11,13-15H,3-7H2,1-2H3/t11-,13+,14+,15+/m1/s1 | |
Source | PubChem | |
URL | https://pubchem.ncbi.nlm.nih.gov | |
Description | Data deposited in or computed by PubChem | |
InChI Key |
SVAPNGMAOHQQFJ-UNQGMJICSA-N | |
Source | PubChem | |
URL | https://pubchem.ncbi.nlm.nih.gov | |
Description | Data deposited in or computed by PubChem | |
Canonical SMILES |
CC1CCC(C2C1CCC(=C2)C)C(=C)C=O | |
Source | PubChem | |
URL | https://pubchem.ncbi.nlm.nih.gov | |
Description | Data deposited in or computed by PubChem | |
Isomeric SMILES |
C[C@@H]1CC[C@H]([C@@H]2[C@H]1CCC(=C2)C)C(=C)C=O | |
Source | PubChem | |
URL | https://pubchem.ncbi.nlm.nih.gov | |
Description | Data deposited in or computed by PubChem | |
Molecular Formula |
C15H22O | |
Source | PubChem | |
URL | https://pubchem.ncbi.nlm.nih.gov | |
Description | Data deposited in or computed by PubChem | |
Molecular Weight |
218.33 g/mol | |
Source | PubChem | |
URL | https://pubchem.ncbi.nlm.nih.gov | |
Description | Data deposited in or computed by PubChem | |
Product Name |
Artemisinic aldehyde |
Retrosynthesis Analysis
AI-Powered Synthesis Planning: Our tool employs the Template_relevance Pistachio, Template_relevance Bkms_metabolic, Template_relevance Pistachio_ringbreaker, Template_relevance Reaxys, Template_relevance Reaxys_biocatalysis model, leveraging a vast database of chemical reactions to predict feasible synthetic routes.
One-Step Synthesis Focus: Specifically designed for one-step synthesis, it provides concise and direct routes for your target compounds, streamlining the synthesis process.
Accurate Predictions: Utilizing the extensive PISTACHIO, BKMS_METABOLIC, PISTACHIO_RINGBREAKER, REAXYS, REAXYS_BIOCATALYSIS database, our tool offers high-accuracy predictions, reflecting the latest in chemical research and data.
Strategy Settings
Precursor scoring | Relevance Heuristic |
---|---|
Min. plausibility | 0.01 |
Model | Template_relevance |
Template Set | Pistachio/Bkms_metabolic/Pistachio_ringbreaker/Reaxys/Reaxys_biocatalysis |
Top-N result to add to graph | 6 |
Feasible Synthetic Routes
Q & A
Q1: What is the role of artemisinic aldehyde in artemisinin biosynthesis?
A1: Artemisinic aldehyde is a crucial branch point in the artemisinin biosynthetic pathway [, , ]. It can be converted to artemisinic acid by amorphadiene oxidase (AMO), leading to the production of arteannuin B. Alternatively, artemisinic aldehyde Δ11(13) reductase (DBR2) can reduce it to dihydroartemisinic aldehyde, which is further oxidized to dihydroartemisinic acid (DHAA), the precursor of artemisinin [, ].
Q2: Can the production of artemisinin be enhanced by manipulating the enzymes involved in artemisinic aldehyde metabolism?
A2: Yes, research suggests that manipulating the expression of enzymes like DBR2 can influence artemisinin yield. Overexpressing DBR2 in Artemisia annua has been shown to increase the levels of both artemisinin and its precursor DHAA [, , ]. Inhibiting branching pathways that compete with artemisinin biosynthesis, such as those leading to β-caryophyllene or β-farnesene, can also enhance artemisinin production [].
Q3: Is the activity of DBR2 influenced by factors beyond its expression level?
A3: Recent studies have revealed that variations in the promoter region of the DBR2 gene can significantly affect its expression level and, consequently, artemisinin yield in different chemotypes of Artemisia annua [, , ]. This finding highlights the importance of considering genetic factors beyond gene expression when engineering artemisinin biosynthesis.
Q4: Can artemisinin or its precursors be produced in organisms other than Artemisia annua?
A4: Research shows promising results for heterologous production. For instance, expressing the artemisinin pathway genes in yeast (Saccharomyces cerevisiae) has enabled the production of DHAA [, ]. Similarly, introducing these genes into tobacco plants resulted in the accumulation of artemisinin precursors, including artemisinic alcohol and dihydroartemisinic alcohol [, ].
Q5: What challenges remain in engineering artemisinin biosynthesis in heterologous hosts?
A5: While promising, heterologous production faces hurdles. In tobacco, for example, the pathway seems to favor the reduction of aldehydes to alcohols rather than their oxidation to acids, limiting DHAA accumulation []. Additionally, glycosylation and glutathione conjugation of pathway intermediates can divert metabolic flux away from DHAA [].
Q6: What is the function of artemisinic aldehyde Δ11(13) reductase (DBR2)?
A6: DBR2, found in the glandular trichomes of Artemisia annua, catalyzes the reduction of the Δ11(13) double bond in artemisinic aldehyde, yielding dihydroartemisinic aldehyde [, ]. This reduction is a critical step in directing the pathway towards artemisinin production.
Q7: Are there DBR2 homologs in other Artemisia species?
A7: While other Artemisia species don't produce artemisinin, research has identified a DBR2 homolog (abDBR2) in Artemisia absinthium [, ]. This homolog exhibits comparable activity to A. annua DBR2 in converting artemisinic aldehyde to dihydroartemisinic aldehyde. Notably, dihydroartemisinic aldehyde was detected in A. absinthium leaves fed with artemisinic aldehyde, suggesting active abDBR2 in planta [].
Q8: What are the implications of finding functional DBR2 homologs in non-artemisinin producing plants?
A8: The presence of functional DBR2 homologs in species like A. absinthium suggests these plants might possess a partial artemisinin-producing ability [, ]. This discovery opens avenues for exploring the use of metabolic engineering to induce artemisinin production in these alternative hosts.
Q9: What are the phytochemical differences between high- and low-artemisinin producing (HAP and LAP) chemotypes of Artemisia annua?
A10: Analysis of HAP and LAP chemotypes revealed distinct terpenoid profiles []. The LAP chemotype, characterized by low DBR2 expression, accumulates higher levels of artemisinic acid, arteannuin B and other sesquiterpenes unsaturated at the 11,13-position []. In contrast, the HAP chemotype, with higher DBR2 expression, shows higher levels of DHAA, artemisinin, and other sesquiterpenes saturated at the 11,13-position []. This difference highlights the role of DBR2 in controlling the metabolic flux towards artemisinin biosynthesis.
Q10: What are some future research directions in the study of artemisinic aldehyde?
A10: Future research could focus on:
- Exploring transport and sequestration: Understanding the mechanisms involved in transporting and sequestering artemisinic aldehyde and its derivatives within the cell and to the apoplast [].
- Investigating the role of transcription factors: Identifying and manipulating transcription factors that regulate DBR2 and other genes in the artemisinin pathway [, ].
Haftungsausschluss und Informationen zu In-Vitro-Forschungsprodukten
Bitte beachten Sie, dass alle Artikel und Produktinformationen, die auf BenchChem präsentiert werden, ausschließlich zu Informationszwecken bestimmt sind. Die auf BenchChem zum Kauf angebotenen Produkte sind speziell für In-vitro-Studien konzipiert, die außerhalb lebender Organismen durchgeführt werden. In-vitro-Studien, abgeleitet von dem lateinischen Begriff "in Glas", beinhalten Experimente, die in kontrollierten Laborumgebungen unter Verwendung von Zellen oder Geweben durchgeführt werden. Es ist wichtig zu beachten, dass diese Produkte nicht als Arzneimittel oder Medikamente eingestuft sind und keine Zulassung der FDA für die Vorbeugung, Behandlung oder Heilung von medizinischen Zuständen, Beschwerden oder Krankheiten erhalten haben. Wir müssen betonen, dass jede Form der körperlichen Einführung dieser Produkte in Menschen oder Tiere gesetzlich strikt untersagt ist. Es ist unerlässlich, sich an diese Richtlinien zu halten, um die Einhaltung rechtlicher und ethischer Standards in Forschung und Experiment zu gewährleisten.