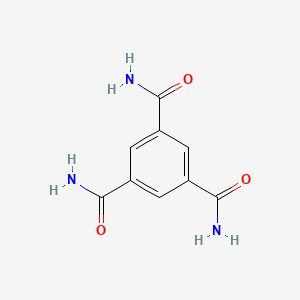
Benzol-1,3,5-tricarboxamid
Übersicht
Beschreibung
Benzene-1,3,5-tricarboxamide (BTA) has gained significant attention in supramolecular chemistry since its first synthesis in 1915. Known for its simple structure and the detailed understanding of its supramolecular self-assembly behavior, BTA serves as a versatile building block in various scientific fields. The self-assembly of BTAs into one-dimensional, nanometer-sized rod-like structures is stabilized by threefold hydrogen bonding, showcasing their potential in nanotechnology, polymer processing, and biomedical applications (Cantekin, de Greef, & Palmans, 2012).
Synthesis Analysis
The synthesis of BTAs involves straightforward methods allowing for the introduction of various functional groups, thereby tailoring their physical and chemical properties. Flexible BTAs, in particular, have been explored as structurally reinforcing supramolecular building blocks in porous coordination polymers, demonstrating the adaptability of their synthesis and the resulting structural resilience (Lynes et al., 2018).
Molecular Structure Analysis
The molecular structure of BTAs is characterized by their ability to form functional soft materials through complementary hydrogen bonding. This unique property is harnessed in the design of carboxylate-terminated BTA derivatives, leading to the formation of polymeric Cd(II) complexes with notable structural resilience and gas adsorption capacities (Lynes et al., 2018).
Chemical Reactions and Properties
BTAs exhibit a wide range of chemical reactions and properties, primarily due to their ability to engage in hydrogen bonding and π-π interactions. These interactions not only facilitate their self-assembly into higher-order structures but also impact their reactivity and interaction with other molecules. The synthesis of asymmetrically substituted BTAs, for example, demonstrates the influence of molecular structure on their liquid-crystalline properties and aggregation behavior (Stals et al., 2009).
Physical Properties Analysis
The physical properties of BTAs, such as their morphological and thermal characteristics, can be finely tuned by minor modifications in their molecular structure. This tunability is evident in the formation of gels and crystalline phases by carboxylic acid and ester derivatives of BTAs, respectively. Such modifications influence the self-assembly behavior of BTAs, leading to diverse structural formations with specific thermotropic phase transitions (Lynes et al., 2017).
Chemical Properties Analysis
The chemical properties of BTAs are deeply influenced by their supramolecular assembly, which is governed by hydrogen bonding and the nature of their substituents. The assembly properties of BTAs can be modulated to form either helical rods or dimeric structures, impacting their functionality in various applications. This modulation is achieved through the strategic design of BTA derivatives, highlighting the relationship between molecular structure and supramolecular assembly (Desmarchelier et al., 2016).
Wissenschaftliche Forschungsanwendungen
Supramolekulare Chemie
BTA wurde als vielseitiges Ordnungselement für die supramolekulare Chemie identifiziert . Seine einfache Struktur und breite Zugänglichkeit, kombiniert mit einem detaillierten Verständnis seines supramolekularen Selbstassemblierungsverhaltens, ermöglichen die vollständige Nutzung dieses vielseitigen, supramolekularen Bausteins .
Nanotechnologie
Im Bereich der Nanotechnologie werden BTAs aufgrund ihrer Fähigkeit, sich zu eindimensionalen, nanometergroßen stäbchenförmigen Strukturen selbst zu assemblieren, die durch dreifache H-Bindung stabilisiert sind, eingesetzt . Diese Eigenschaft macht sie ideal für die Herstellung von Nanostrukturen mit spezifischen Eigenschaften.
Polymerverarbeitung
BTAs werden auch in der Polymerverarbeitung eingesetzt. Ihr Selbstassemblierungsverhalten ermöglicht es, sie als Bausteine bei der Herstellung komplexer Polymerstrukturen zu verwenden . Dies kann zu Polymeren mit einzigartigen Eigenschaften führen, die für bestimmte Anwendungen maßgeschneidert werden können.
Biomedizinische Anwendungen
Die multivalente Natur von BTAs treibt Anwendungen im biomedizinischen Bereich voran . Sie können verwendet werden, um funktionelle mehrkomponentige Biomaterialien zu erzeugen . Ihre Morphologien und ihr intrinsisches dynamisches Verhalten ahmen faserige Strukturen nach, die in der Natur vorkommen, was sie für biomedizinische Anwendungen einzigartig geeignet macht .
Funktionelle mehrkomponentige Biomaterialien
BTAs haben in den letzten Jahren viel Aufmerksamkeit erregt, da sie sich hervorragend eignen, um funktionelle mehrkomponentige Biomaterialien zu erzeugen . Ihre Modularität ermöglicht die Kontrolle der Dichte der Funktionalitäten, die auf der Oberfläche der Fasern präsentiert werden, wenn funktionalisierte BTA-Monomere verwendet werden .
Supramolekularer Polymer
BTAs werden verwendet, um synthetische, wasserverträgliche supramolekulare Polymere herzustellen . Diese Polymere weisen eine Morphologie und ein dynamisches Verhalten auf, das dem von BTA-Homopolymeren ähnelt . Sie können mit einem Fluoreszenzfarbstoff dekoriert werden, um ihre Ko-Assemblierung mit BTAs zu visualisieren .
Wirkmechanismus
Target of Action
Benzene-1,3,5-tricarboxamide (BTA) is a versatile molecule that primarily targets the formation of supramolecular structures . Its simple structure and wide accessibility, combined with a detailed understanding of its supramolecular self-assembly behavior, allow full utilization of this versatile, supramolecular building block in applications ranging from nanotechnology to polymer processing and biomedical applications .
Mode of Action
The mode of action of BTA is primarily through the self-assembly into one-dimensional, nanometer-sized rod-like structures stabilized by threefold H-bonding . This self-assembly is driven by the multivalent nature of BTAs, which is particularly useful in the biomedical field .
Biochemical Pathways
The biochemical pathways affected by BTAs are related to their ability to form functional soft-materials by virtue of their complementary hydrogen bonding . They are explored as structurally reinforcing supramolecular building blocks in porous coordination polymers .
Pharmacokinetics
Their solubility and self-assembly behavior are critical factors influencing their bioavailability . For instance, inversed amide BTAs (iBTAs) form intermolecular hydrogen bonds and assemble into supramolecular polymers, albeit with a slight decrease in water solubility .
Result of Action
The result of BTA’s action is the formation of supramolecular structures that mimic fibrous structures found in nature . These structures can be used to generate functional multicomponent biomaterials . For instance, BTAs have been used to create scaffolds for cartilage and bone regeneration, targeted therapy for atherosclerosis, and the promotion of angiogenesis .
Action Environment
The action of BTAs can be influenced by environmental factors. For example, the dynamic properties of BTAs can be tuned by mixing mono- and bivalent variants . This allows the control of the number of biological recognition motifs presented on the supramolecular structures by mixing in different functional monomers in the desired ratio .
Safety and Hazards
Zukünftige Richtungen
BTAs have attracted a lot of interest in recent years, as they are uniquely suited to generate functional multicomponent biomaterials . Their morphologies and intrinsic dynamic behavior mimic fibrous structures found in nature . Currently, the first commercial applications of BTAs are emerging . The adaptable nature of this multipurpose building block promises a bright future .
Eigenschaften
IUPAC Name |
benzene-1,3,5-tricarboxamide | |
---|---|---|
Source | PubChem | |
URL | https://pubchem.ncbi.nlm.nih.gov | |
Description | Data deposited in or computed by PubChem | |
InChI |
InChI=1S/C9H9N3O3/c10-7(13)4-1-5(8(11)14)3-6(2-4)9(12)15/h1-3H,(H2,10,13)(H2,11,14)(H2,12,15) | |
Source | PubChem | |
URL | https://pubchem.ncbi.nlm.nih.gov | |
Description | Data deposited in or computed by PubChem | |
InChI Key |
WXWQVSOHWXJBDF-UHFFFAOYSA-N | |
Source | PubChem | |
URL | https://pubchem.ncbi.nlm.nih.gov | |
Description | Data deposited in or computed by PubChem | |
Canonical SMILES |
C1=C(C=C(C=C1C(=O)N)C(=O)N)C(=O)N | |
Source | PubChem | |
URL | https://pubchem.ncbi.nlm.nih.gov | |
Description | Data deposited in or computed by PubChem | |
Molecular Formula |
C9H9N3O3 | |
Source | PubChem | |
URL | https://pubchem.ncbi.nlm.nih.gov | |
Description | Data deposited in or computed by PubChem | |
DSSTOX Substance ID |
DTXSID90332281 | |
Record name | 1,3,5-Benzenetricarboxamide | |
Source | EPA DSSTox | |
URL | https://comptox.epa.gov/dashboard/DTXSID90332281 | |
Description | DSSTox provides a high quality public chemistry resource for supporting improved predictive toxicology. | |
Molecular Weight |
207.19 g/mol | |
Source | PubChem | |
URL | https://pubchem.ncbi.nlm.nih.gov | |
Description | Data deposited in or computed by PubChem | |
CAS RN |
60541-32-4 | |
Record name | 1,3,5-Benzenetricarboxamide | |
Source | CAS Common Chemistry | |
URL | https://commonchemistry.cas.org/detail?cas_rn=60541-32-4 | |
Description | CAS Common Chemistry is an open community resource for accessing chemical information. Nearly 500,000 chemical substances from CAS REGISTRY cover areas of community interest, including common and frequently regulated chemicals, and those relevant to high school and undergraduate chemistry classes. This chemical information, curated by our expert scientists, is provided in alignment with our mission as a division of the American Chemical Society. | |
Explanation | The data from CAS Common Chemistry is provided under a CC-BY-NC 4.0 license, unless otherwise stated. | |
Record name | 1,3,5-Benzenetricarboxamide | |
Source | EPA DSSTox | |
URL | https://comptox.epa.gov/dashboard/DTXSID90332281 | |
Description | DSSTox provides a high quality public chemistry resource for supporting improved predictive toxicology. | |
Retrosynthesis Analysis
AI-Powered Synthesis Planning: Our tool employs the Template_relevance Pistachio, Template_relevance Bkms_metabolic, Template_relevance Pistachio_ringbreaker, Template_relevance Reaxys, Template_relevance Reaxys_biocatalysis model, leveraging a vast database of chemical reactions to predict feasible synthetic routes.
One-Step Synthesis Focus: Specifically designed for one-step synthesis, it provides concise and direct routes for your target compounds, streamlining the synthesis process.
Accurate Predictions: Utilizing the extensive PISTACHIO, BKMS_METABOLIC, PISTACHIO_RINGBREAKER, REAXYS, REAXYS_BIOCATALYSIS database, our tool offers high-accuracy predictions, reflecting the latest in chemical research and data.
Strategy Settings
Precursor scoring | Relevance Heuristic |
---|---|
Min. plausibility | 0.01 |
Model | Template_relevance |
Template Set | Pistachio/Bkms_metabolic/Pistachio_ringbreaker/Reaxys/Reaxys_biocatalysis |
Top-N result to add to graph | 6 |
Feasible Synthetic Routes
Q & A
Q1: What is the primary driving force for BTA self-assembly?
A: The primary driving force behind BTA self-assembly is the formation of strong, directional threefold α-helical type intermolecular hydrogen bonds between adjacent BTA molecules. [, , ] This leads to the formation of one-dimensional columnar aggregates, often described as helical stacks or nanorods. [, , , , ]
Q2: How does the chirality of BTA monomers influence the self-assembly process?
A: Introducing a stereogenic center into the alkyl side chains of BTAs leads to a preference for one helical conformation over the other, evident in the strong Cotton effects observed in dilute apolar solutions. [, , , ] This chiral amplification arises from the energetic preference of homochiral interactions over heterochiral ones during assembly.
Q3: What types of higher-order structures can BTAs form?
A3: Beyond one-dimensional aggregates, BTAs can assemble into more complex architectures. For instance, they can form:
- Twisted nanofibers: Driven by interfiber interactions and influenced by the peptide sequence in BTA-peptide conjugates. []
- Organogels: Formed in various nonpolar solvents, exhibiting viscoelastic properties dependent on BTA concentration and solvent type. [, , ]
- Membranes and nanotubes: Observed with carboxylic acid functionalized, water-soluble BTAs, showcasing the system's ability to adopt polymorphic structures. []
Q4: How does solvent polarity affect BTA self-assembly?
A: BTA self-assembly is highly sensitive to solvent polarity. Increasing the solvent polarity disrupts the hydrogen bonding network, leading to a decrease in aggregate stability. [, ] This effect is observed both in dilute solutions and in solid-state materials where BTAs are incorporated into polymers. []
Q5: How do the alkyl side chains of BTAs impact their self-assembly?
A5: The length and branching of alkyl side chains significantly impact BTA self-assembly:
- Chain Length: Increasing the chain length generally enhances the stability of aggregates due to stronger van der Waals interactions between side chains. []
- Branching: Branching can influence the packing arrangements and liquid crystalline behavior. For example, branched 3,7-dimethyloctanoyl chains promote the formation of liquid crystalline phases, while linear chains might favor crystalline structures. []
Q6: Can the hydrogen bonding pattern in BTAs be modified?
A: Yes, modifications to the BTA core can alter the hydrogen bonding pattern. For instance, amino ester-based BTAs exhibit both amide-amide (AA) and amide-carboxylate (AC) hydrogen bonding. [] While AC dimers are more thermodynamically stable, AA interactions are crucial for the formation of extended supramolecular polymers. []
Q7: What are some notable properties of BTA-based materials?
A7: BTA-based materials exhibit a range of interesting properties, including:
- Thermotropic liquid crystallinity: Observed in several BTA derivatives, with the mesophase stability and range influenced by side chain structure and intermolecular interactions. [, ]
- Viscoelasticity: BTA organogels demonstrate viscoelastic behavior, with properties tunable by varying BTA concentration, solvent, and temperature. [, ]
- Polar switching: Hydrogen-bonded hexagonal columnar liquid crystal phases formed by BTAs can be aligned by electric fields, displaying polar switching behavior with potential applications in electronic devices. []
- Fluorescence: BTA derivatives incorporating fluorophores like cyanostilbene exhibit aggregation-induced emission enhancement (AIEE), with fluorescence properties tunable by controlling the self-assembly process. []
Q8: What are the potential applications of BTAs in materials science?
A8: The unique self-assembly and stimuli-responsive properties of BTAs make them attractive candidates for various applications, including:
- Electro-optic devices: BTAs that form liquid crystalline phases with polar switching behavior show promise for use in displays, sensors, and other electro-optic applications. [, ]
- Organogels and soft materials: BTA-based organogels, with their tunable viscoelastic properties, can be used in areas like drug delivery, cosmetics, and sensors. [, ]
- Biomimetic materials: The fibrous nature of BTA assemblies and their ability to incorporate biomolecules make them promising for developing biocompatible scaffolds for tissue engineering and drug delivery. [, ]
Q9: How have computational methods been used to study BTA self-assembly?
A9: Computational chemistry plays a crucial role in understanding and predicting BTA self-assembly. Techniques like:
- Density functional theory (DFT): Used to investigate the strength and geometry of hydrogen bonding interactions, providing insights into the driving forces behind BTA self-assembly and the impact of structural modifications. [, , , ]
- Molecular dynamics (MD) simulations: Employed to simulate the self-assembly process in realistic solvents, providing information on aggregate stability, dynamics, and the influence of solvent on assembly. [, , ]
Q10: What is the significance of the cooperativity factor (σ) in BTA self-assembly?
A: The cooperativity factor quantifies the extent of cooperativity in the self-assembly process. BTAs exhibit a wide range of σ values, from isodesmic (σ = 1) to highly cooperative (σ < 10-6). [] Understanding the relationship between BTA structure and σ allows for the rational design of BTA derivatives with tailored self-assembly properties. []
Q11: How does the amide connectivity in BTAs influence their properties?
A: The amide connectivity significantly affects BTA self-assembly. While conventionally synthesized BTAs have carbonyl (C=O)-centered amides, N-centered BTAs (N-BTAs) show weaker aggregation and less pronounced chiral amplification. [] DFT calculations revealed that this difference stems from the lower interaction energy between monomeric units in N-BTA supramolecular polymers. []
Haftungsausschluss und Informationen zu In-Vitro-Forschungsprodukten
Bitte beachten Sie, dass alle Artikel und Produktinformationen, die auf BenchChem präsentiert werden, ausschließlich zu Informationszwecken bestimmt sind. Die auf BenchChem zum Kauf angebotenen Produkte sind speziell für In-vitro-Studien konzipiert, die außerhalb lebender Organismen durchgeführt werden. In-vitro-Studien, abgeleitet von dem lateinischen Begriff "in Glas", beinhalten Experimente, die in kontrollierten Laborumgebungen unter Verwendung von Zellen oder Geweben durchgeführt werden. Es ist wichtig zu beachten, dass diese Produkte nicht als Arzneimittel oder Medikamente eingestuft sind und keine Zulassung der FDA für die Vorbeugung, Behandlung oder Heilung von medizinischen Zuständen, Beschwerden oder Krankheiten erhalten haben. Wir müssen betonen, dass jede Form der körperlichen Einführung dieser Produkte in Menschen oder Tiere gesetzlich strikt untersagt ist. Es ist unerlässlich, sich an diese Richtlinien zu halten, um die Einhaltung rechtlicher und ethischer Standards in Forschung und Experiment zu gewährleisten.